![]() |
Drug Target Insights 2024; 18: 70-77 ISSN 1177-3928 | DOI: 10.33393/dti.2024.3177 ORIGINAL RESEARCH ARTICLE |
![]() |
Enhancement of apoptosis in Caco-2, Hep-G2, and HT29 cancer cell lines following exposure to Toxoplasma gondii peptides
ABSTRACT
Objective: Cancer or neoplasm is a cosmopolitan catastrophe that results in more than 20 million new cases and 10 million deaths every year. Some factors lead to carcinogenesis like infectious diseases. Parasites like Toxoplasma gondii, by its components, could modulate the cancer system by inducing apoptosis. The objective of this investigation is to assess the potential of peptides derived from T. gondii in combating cancer by examining their effects on Caco-2, Hep-G2, and HT29 cell lines.
Materials and methods: Candidate peptide by its similarity to anticancer compounds was predicted through the computer-based analysis/platform. The impact of the peptide on cell viability, cell proliferation, and gene expression was evaluated through the utilization of MTT assay, flow cytometry, and real-time polymerase chain reaction (PCR) methodologies.
Results: The cell viability rate exhibited a significant decrease (p < 0.001) across all cell lines when exposed to a concentration of ≤160 μg. Within the 48-hour timeframe, the half maximal inhibitory concentration (IC50) for HT29 and Hep-G2 cell lines was determined to be 107.2 and 140.6 μg/mL, respectively. Notably, a marked decrease in the expression levels of Bcl2 and APAF1 genes was observed in both the Hep-G2 and HT29 cell lines.
Conclusion: These findings indicate that the T. gondii peptide affected cancer cell mortality and led to changes in the expression of genes associated with apoptosis.
Keywords: Anticancer, Neoplasm, Parasite, Peptides, Real-time PCR, Toxoplasmosis
Received: June 24, 2024
Accepted: July 11, 2024
Published online: September 30, 2024
Corresponding author:
Javid Sadraei
email: Sadraeij@modares.ac.ir
Drug Target Insights - ISSN 1177-3928 - www.aboutscience.eu/dti
© 2024 The Authors. This article is published by AboutScience and licensed under Creative Commons Attribution-NonCommercial 4.0 International (CC BY-NC 4.0).
Commercial use is not permitted and is subject to Publisher’s permissions. Full information is available at www.aboutscience.eu
Introduction
Toxoplasma gondii is the most widespread protozoan parasite that gained its reputation by seropositive analysis. It has been found that more than 30%-50% of the world’s population is positive for T. gondii (1). Felids are known to be the definitive host in which the sexual stage takes place. Terrestrial and aquatic mammals as well as birds act as intermediate hosts during the asexual stage (2).
Three developmental stages have been distinguished to infect the cell: (i) tachyzoite, which rapidly multiplies and occurs in the acute phase of the infection, (ii) bradyzoite, a form of slow multiplication that characterizes the chronic phase, and (iii) sporozoites, which are distinctive of the sexual stage in felids and found in oocysts in feline feces (3).
Various pathways have been reported for the infection of intermediate hosts: ingestion of oocysts-contaminated fruits, vegetables, or water, consumption of raw or uncooked meat containing tissue cysts, congenital transmission, blood transfusion, and organ transplantation. Although transmission through the ingestion of non-pasteurized milk or milk products has been documented, it is not common (4). It is obvious that felines as definitive hosts can become infected through carnivorous behavior or ingestion of sporulated oocysts (5).
Cancer is referred as a composite of diseases acquired by the development of neoplastic cells (6). It contributes symptoms like eluding growth suppressors, empowering proliferative signaling, withstanding cell death, promoting the ability to replicate indefinitely, activating angiogenesis, prompting invasion and metastasis, genome vulnerability, reconstructing energy metabolism, and evading destruction by the immune system as well (7). There are more than 20 million newly diagnosed cases of cancer and almost 10 million deaths attributed to this disease every year (8). Multiple factors play a pivotal role in causing carcinogenesis like lifestyle, genes, and the microenvironment. Moreover, it is widely recognized that infectious diseases play a significant role in the development of various types of cancers. These diseases are responsible for approximately 25%-50% of all cancer cases (9). Notably, viral infections such as papilloma virus have been linked to cervical cancer, while bacterial infections like Helicobacter pylori have been associated with gastric cancer. Additionally, parasitic infections, including Schistosoma haematobium, have been found to be connected to urinary bladder cancer. The strong correlation between infectious diseases and cancer underscores the importance of preventive measures and early detection in reducing the burden of these malignancies. In this way, some parasites act as the inducers or promoters of the cancers and others inhibit the factors that could modulate tumorigenesis. So far, investigations show that components of parasites are able to modulate the cancer system by inducing apoptosis (10). Studies have shown that T. gondii is able to break tumor immune tolerance and arouse potent CD8+ T-cell immunity (11). In the current study, the effect of T. gondii-synthesized excretory-secretory compound in cell growth factors and inducing apoptosis was investigated.
Materials and methods
Synthesis of peptide
Out of the acknowledged proteomes of T. gondii excretory-secretory antigens, the QLEDAVSAVASVVQDE amino acid sequence belonging to part GRA1 was selected. It is noteworthy that this sequence has the most similarity to the other anticancer agents (≈91%) by the analysis done in association with the anticancer peptide database: CancerPPD site (Online). Following analysis of the nominated sequence and confirmation of its anticancer potential, ElabScience was proposed for peptide synthesis (United States, Lot No: YZIGY9RHUD) with a purity of over 97.5% and a molecular weight of 2,425.5. As per the guidelines provided by the manufacturer, by applying ultra-pure water, the synthesized peptide underwent dilution and was subsequently prepared in various concentrations to evaluate cell viability and perform molecular assays.
Anticancer assays in vitro
Cell culture and treatments
The Iranian Biological Resource Center (IBRC) provided the Caco-2, Hep-G2, and HT29 cell lines, which are human gastric, colon, and liver cancer cell lines, respectively. These cell lines were cultured in Dulbecco’s modified Eagle’s medium (DMEM)/F12, supplemented with 10% fetal bovine serum (FBS; Gibco, Thermo Fisher Scientific) and 2 mmol/L L-glutamine (Bio Idea Co, Iran). The growth medium for all cell lines consisted of DMEM/F12 supplemented with GlutaMAX, NaHCO3, and 15 mM HEPES. To ensure optimal conditions for cell growth, the cells were incubated at a temperature of 37°C in a humidified atmosphere consisting of 5% carbon dioxide (12).
MTT assay for cell viability detection
After achieving a confluence of 90%, the cells were seeded into the wells using microscopic counting after being stained with trypan blue. MTT assay was administered in 96-well culture plates for cell viability. Each well was seeded with approximately 2 × 104 cells in 200 μL of DMEM medium. The plates were then incubated at 37°C and 5% CO2 for 24 hours to ensure proper adherence of the cells to the wells of the plate. Subsequently, Hep-G2, HT29, and Caco-2 cells were exposed to elevating concentrations of T. gondii peptide (40, 80, and 160 μg/mL) and incubated for 24 and 48 hours in the 96-well culture plates. The growth of the cells was assessed based on the activity of mitochondrial enzymes determined in the MTT assay.
Following 24- and 48-hour exposure of cell lines to the peptides, 20 μL volume of 5 μg/mL MTT solutions were added to the wells, and the plates were then incubated at 37°C for an additional 4 hours. Subsequently, the wells were emptied and 200 μL of dimethyl sulfoxide (DMSO) was added to all wells; in this specific approach, living cells contain NAD(P)H-dependent oxidoreductase enzymes that facilitate the conversion of the MTT reagent into formazan crystalline product, leading to purple color. The more viable cells and metabolic activity, the more intensity of purple color (13). To measure the optical density (OD) of the wells, a microplate spectrophotometer (BioTek-ELX800, United States) was employed at 570 nm, both 24 and 48 hours after exposure. Each concentration and cell lines were subjected to triplicate experiments to ensure the accuracy and reliability of the results.
Bcl2, APAF1 gene expression analysis
The evaluation of gene expression was conducted using the SYBR green-based quantitative real-time polymerase chain reaction (PCR) technique. In order to measure the messenger ribonucleic acid (mRNA) expression of the Bcl2 and APAF1 genes, the genomic content (total RNA) was extracted from all cultured cell lines. QIAzol RNA (Qiagen, United States) was utilized for this purpose, following the manufacturer’s protocol. The nanodrop spectrophotometer (Thermo Scientific™ NanoDrop 2000c spectrophotometer) was used to evaluate the quantity, quality, and optimal concentration of the collected RNAs at a 260/280 nm ratio. Following this, the RNAs were transformed into complementary deoxyribonucleic acid (cDNA) by reverse transcription, utilizing RT-specific primers and glyceraldehyde 3-phosphate dehydrogenase (GAPDH) as a reference gene for data normalization (Tab. 1). A LightCycler® 96 thermal cycler (Roche, Germany) was used to conduct real-time reverse transcription (RT)-PCR. Within each reaction, a 20 μL mixture was prepared, containing 8 μL of SYBR Green I Master mix, 1 μL of cDNA serving as the template, 8 μL of nuclease-free distilled water, and 10 pmol of each primer. In the amplification program, the initial denaturation step was performed at a temperature of 95°C for a duration of 8 minutes. This was followed by 40-45 cycles of denaturation at 95°C for 10 seconds, annealing at 57-62°C for 5 seconds, and extension at 72°C for 20 seconds (14,15).
Apoptosis analysis
Apoptosis evaluation was conducted using the Annexin V/PI Apoptosis detection kit (cat. no. AnxF100PI, MabTag GmbH) following peptide treatments. A 6-well plate was utilized, with each well containing a volume of 2.5 mL cell suspension at a density of 3 × 105 cells/mL. Following 24-hour cultures of all three kinds of cells in 200 μM of the peptide, in accordance with the procedures outlined in the preceding section, the cells underwent the specified treatment. The negative control group received an equivalent amount of phosphate-buffered saline (PBS). After the incubation period, the cells were collected and underwent two rounds of washing with cold PBS at a pH of 7.4. Subsequently, the cells were suspended in annexin-binding buffer and exposed to 5 μL of fluorescein isothiocyanate (FITC)-Annexin V and 5 μL of propidium iodide (PI). The samples were thoroughly mixed and incubated in darkness at a temperature of 25°C for a duration of 15 minutes. The stained cells were then analyzed using a Sysmex CyFlow Space flow cytometer. The fluorescence emitted by the cells was measured at wavelengths of 495 and 519 nm following excitation at 488 nm (16).
Statistical analysis
In the current investigation, all experiments were repeated in triplicate. Statistical analysis was conducted to compare and evaluate the treated groups in relation to both each other and the control group. The comparative Ct (ΔΔCt) method was employed to statistically analyze the data. Prior to analysis, the real-time PCR findings underwent preprocessing. Subsequently, the expression levels of selected genes (Bcl2, APAF1) among the study groups were evaluated using Kruskal-Wallis and Mann-Whitney U-tests. A p-value <0.05 was deemed to be statistically significant. All statistical analyses were assessed using GraphPad Prism v 6.1 software.
Results
Mortality and proliferation alter under T. gondii peptide impact in all cell lines
In each cell line, the effect of peptides on cell viability, mortality, and proliferation was examined at 24 and 48 hours at escalating concentrations (Fig. 1). A direct correlation was discovered between the rise in concentration and the rise in mortality and interpreted as dose-dependent manner but wasn’t time dependent. At concentrations of 40, 80, and 160 μg, the cell viability rate exhibited a significant decrease across all cell lines. After 24 hours, the cell lines demonstrated the most significant impact when exposed to concentrations of 160 μg (p < 0.001). Like the earlier pattern, after 48 hours of peptide exposure, cell viability declined at 40, 80, and 160 μg concentrations in contrast to accumulative doses. In Caco-2 cells, in comparison with the control group, a significant mortality induction was experienced in the concentration of 160 μg (p < 0.001). The same significant mortality induction was found in Hep-G2 cell line at the concentration of 160 μg at 48 hours exposure. But in HT29 cell line this significant mortality induction started at 40 μg concentration. Cell viability rates were assessed at various concentrations based on the duration of each cell line. As depicted in Figure 1, it was observed that the impact of the peptide on cancer cells differed depending on the dosage administered.
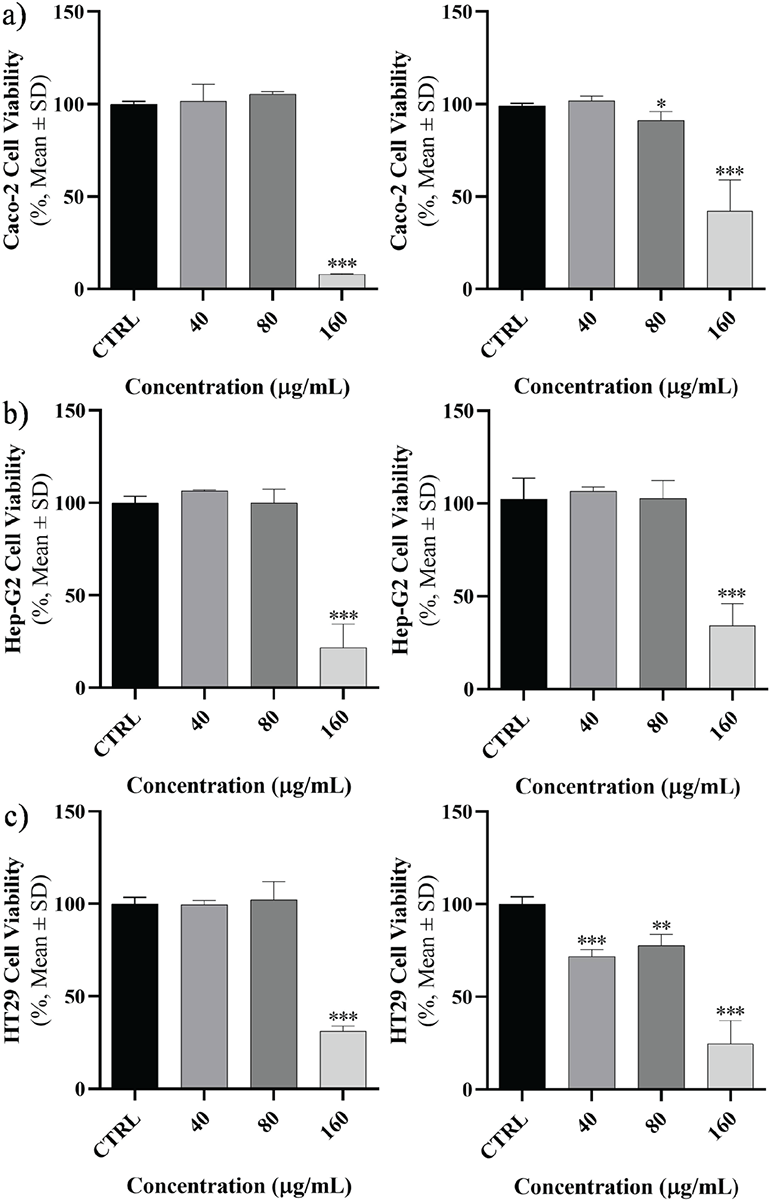
FIGURE 1 - The cell viability of cancer cells. A) Caco-2, B) Hep-G2, and C) HT29 were assessed after 24 (left) and 48 hours (right) exposure to specific concentrations of Toxoplasma gondii-derived peptide (*p <0.05; **p <0.01; ***p <0.001). SD = standard deviation.
The cell viability rate in each cell line was compared at the aforementioned concentrations, which have been provided in Figure 1. Briefly, a significant mortality induction in Caco-2 was started in 160 and 80 μg at 24 and 48 hours, respectively. For Hep-G2, the significant mortality induction was in 160 μg at 24 and 48 hours. This situation was observed in 160 and 40 μg at 24 and 48 hours, respectively.
The IC50 of the excretory-secretory peptide of T. gondii
Based on the results obtained from the MTT assay, the IC50 values for each cell line were determined after exposure to the peptide at both 24 and 48 hours. At the conclusion of the 24-hour period, the Caco-2 cell line exhibited the highest IC50 concentration (149.6 μg/mL), while the Hep-G2 cell line displayed the lowest IC50 concentration (126.8 μg/mL). Furthermore, during the 48-hour timeframe, the HT29 cell line demonstrated the lowest IC50 concentration (107.2 μg/mL), whereas the Hep-G2 cell line exhibited the highest IC50 concentration (140.6 μg/mL) (Fig. 2).
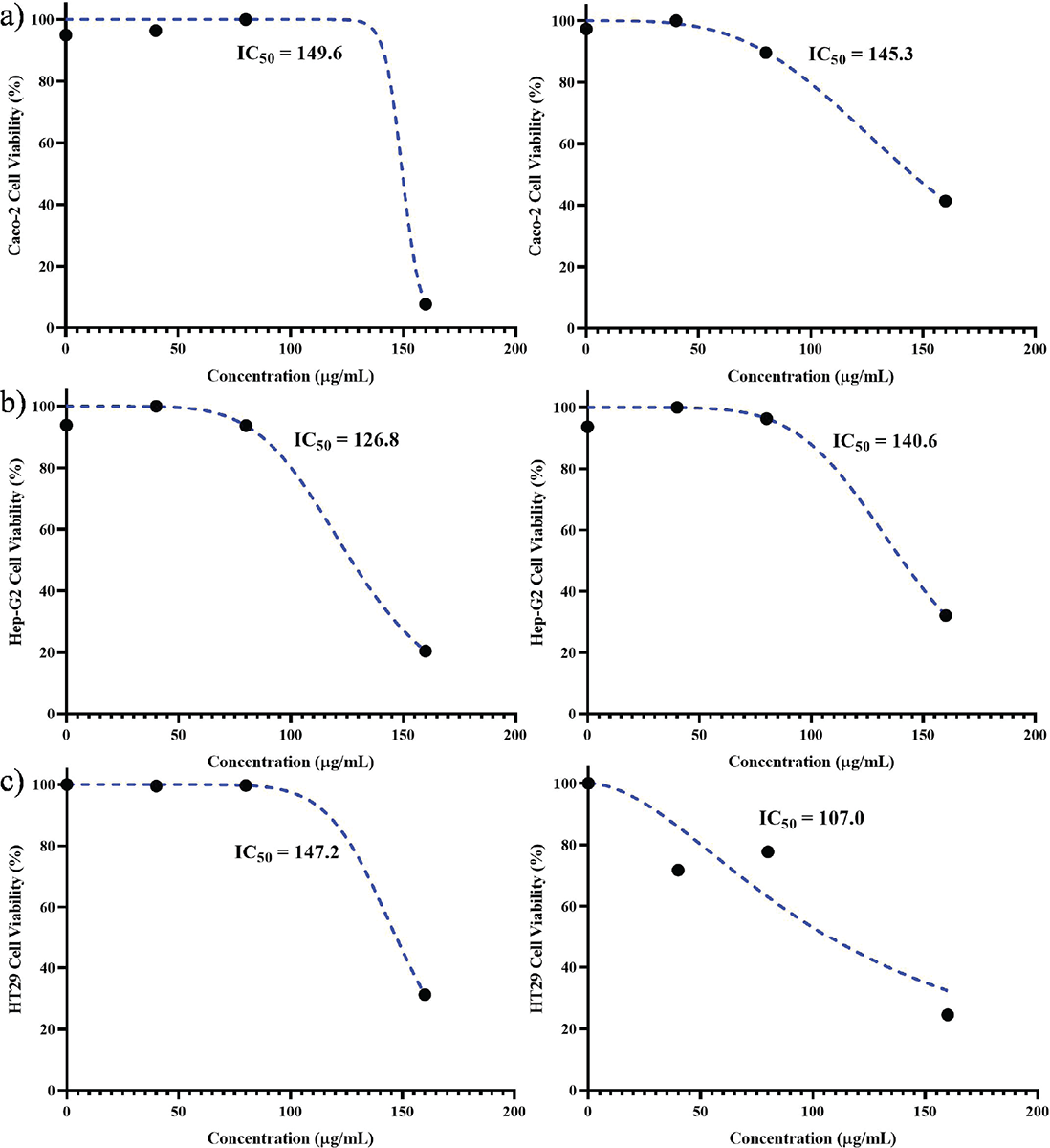
FIGURE 2 - IC50 values of Toxoplasma gondii peptide in cell lines after 24 (left) and 48 hours (right): A) Caco-2, B) Hep-G2, and C) HT29. The highest and lowest values after 24 hours were found in Caco-2 and Hep-G2 cell lines (149.6 and 126.8 µg/mL, respectively) and the highest and lowest values after 48 hours were 150.3 and 107 µg/mL for Caco-2 and HT29 cell lines.
Apoptosis-related gene expression levels
The mRNA levels of Bcl2 and APAF1 were evaluated in three types of cancer cells at exposed concentrations close to IC50 by using real-time PCR and results were reported as a fold change. Evaluated expression levels of Bcl2 and APAF1 genes are shown in Figure 3. In fact, the Caco-2 cell line showed an increase in gene expression level of Bcl2 and non-elevated expression level in APAF1 gene. A notable decline in the expression levels of Bcl2 and APAF1 genes was detected in Hep-G2 and HT29 cell lines (Fig. 3).
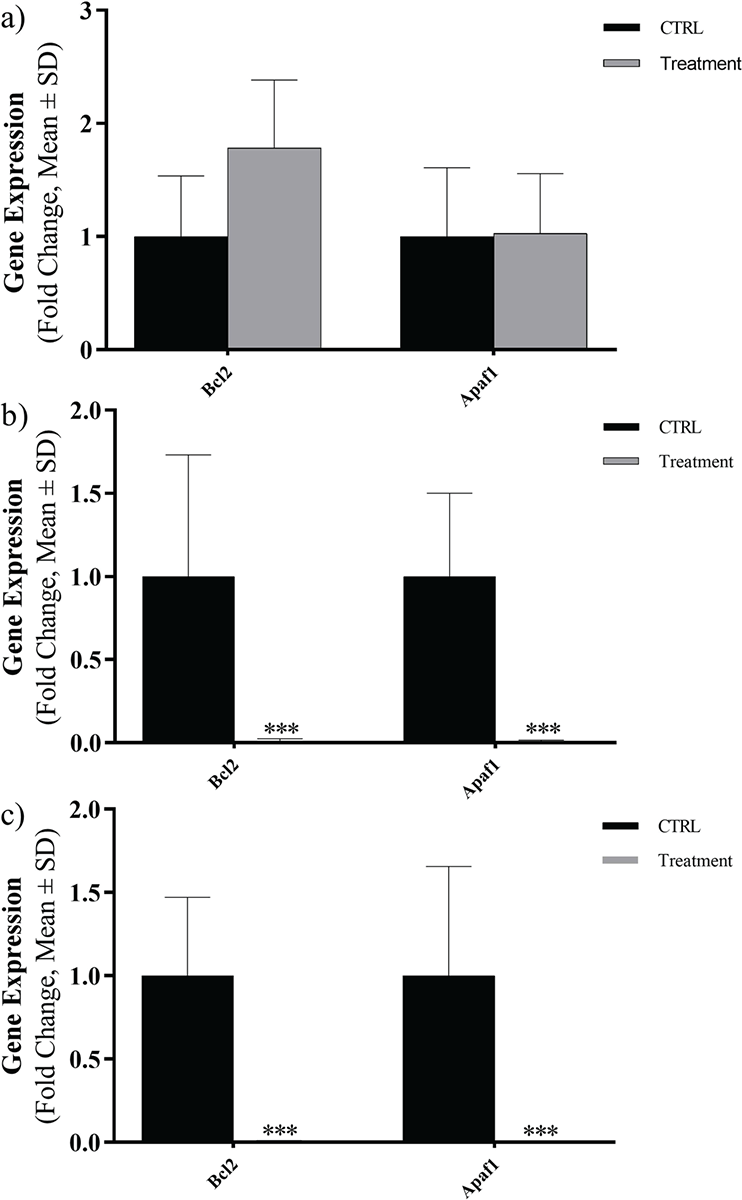
FIGURE 3 - Expression levels of Bcl2 and APAF1 in three cancer cell lines exposed to Toxoplasma gondii peptide in terms of fold change: A) Caco-2, B) Hep-G2, C) HT29 (ns, not significant; *p <0.05; **p <0.01; ***p <0.001; ****p <0.0001). SD = standard deviation.
Flow cytometry
The apoptotic effect was examined on Caco-2, Hep-G2, and HT29 cell lines following treatment with the peptide and subsequent staining with annexin V and PI (Fig. 4). After a 24-hour exposure to the peptide, a noteworthy rise in apoptosis was detected across all cell lines (p = 0.005). Notably, the highest level of apoptosis was observed in HT29 cells, reaching a rate of 32% (Fig. 5).
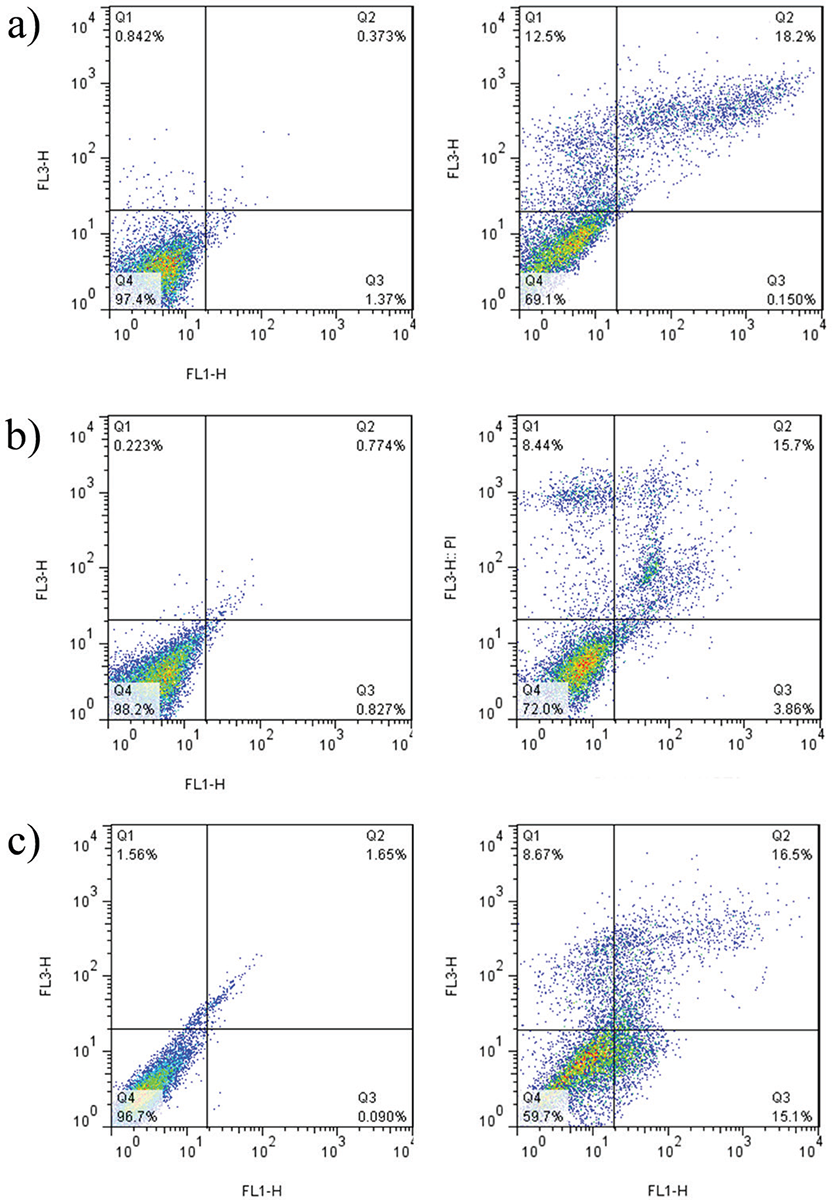
FIGURE 4 - The distribution of apoptotic cells stained with annexin V-FITC/PI in dual parametric dot plots of PI fluorescence (Y-axis) vs. annexin V-FITC fluorescence (X-axis). Dot plots represent cancerous cell lines (A) Caco-2, B) Hep-G2, and C) HT29). The highest rate of apoptosis belongs to HT29 with 32%. FITC = fluorescein isothiocyanate; PI = propidium iodide.
Discussion
In the current investigation, we demonstrated the anticancer effect of candidate Toxoplasma excretory-secretory antigen on three cancerous cell lines: Caco-2, Hep-G2, and HT29. Currently, researchers are still exploring different ways to treat cancer using natural substances instead of chemicals. Besides studying plant compounds, researchers are also assessing the effectiveness of different other sources in inhibiting the growth of cancer cells. Sometimes, it is troublesome to anticipate events of antagonistic impacts from chemotherapeutic drugs amid treatment (17). Cancer cells can become resistant to drugs while receiving chemotherapy. So, to get the same effect in killing tumors, more medicine is needed than what was given initially. Frequently, higher dosage administration creates a higher possibility of side effects in patients (18). Therefore, taking a blend of drugs with various mechanisms could synergistically facilitate therapeutic efficiency (19).
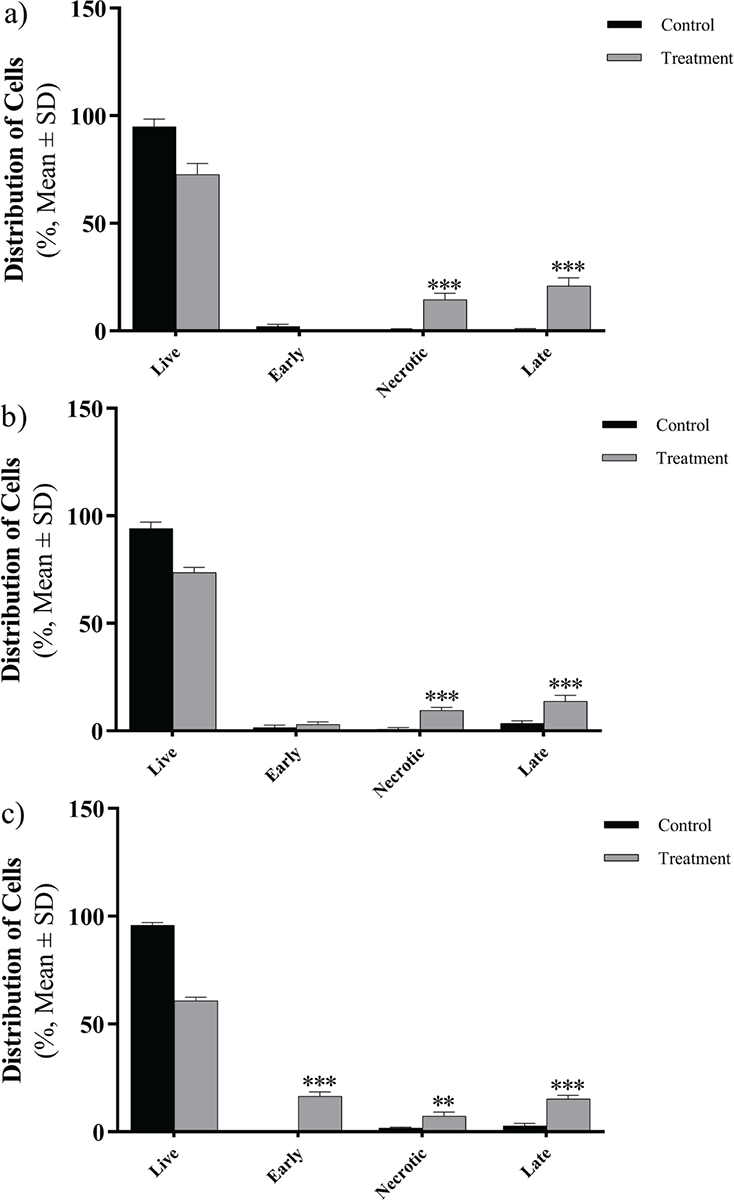
FIGURE 5 - The effects of Toxoplasma gondii peptide on the viability of three cancer cell lines (A) Caco-2, B) Hep-G2, and C) HT29) show a significant increase in induction in comparison to the control. The error bars represent mean ± SD of the triplicate measurements (*p <0.05; **p <0.01; ***p <0.001; ****p <0.0001). SD = standard deviation.
One of these mechanisms could be addressing live organism compounds that have a long relationship with humans and successfully adapted to the human body: parasites. Some studies were conducted by applying protozoa and helminths to improve immunotherapies concentrating against tumors aiming to empower the antitumor immune response and therefore eliminate the progressing neoplasm (7). The pioneer protozoan in uncovering antitumor effects was Trypanosoma cruzi, as affected patients showed no symptoms of colon cancer despite having a tumorigenic process (20). Investigations have revealed that some molecules on the surface of the parasite can induce the production of antibodies that diagnose neoplastic cells due to the antigenic similarity between them, or they could be activators of cells involved in the process of cancer cell recognition. T. gondii itself can trigger a cascade that starts with the induction of interleukin (IL)-12 production and continues with stimulating natural killer (NK) cells and T cells for producing interferon gamma (IFN-γ) and modifying the spread of CD8 T lymphocytes and their cytotoxic capability and finally elevating antitumor immune response (21). The ability of Toxoplasma to modify its host immune response in several studies makes addressing this parasite as a potent inducer of antitumor responses (11).
Our investigation shows that parasite-derived peptide could induce apoptosis in cancer cell lines, which was in line with the lately done study by Bahadory et al (10). According to this study, Toxocara canis excretory-secretory Troponin protein peptide (ES TPP) could successfully alter the expression of apoptotic involved factors. Peptides have characteristically gained more attention in researches due to their advantages; first of all, peptides are the functional piece of natural proteins that can exhibit innumerable biological functions and offer higher selectivity and potency in comparison to ordinary small molecule drugs. Secondly, they could be simply manufactured by chemically solid-phase synthesis. Lastly, possessing amide backbone makes the peptides to be fundamentally biodegradable, which may minimize the side effects (22).
Given that the peptide was synthesized applying computer database, high concentration of peptides, regardless of online prediction, is needed to kill the cells or induce cell death. Scientists suggest that use of nanoparticles, adjuvants, and improving target cell delivery system are means for optimizing compounds with anticancer potential (23). On the basis of attained results, the applied peptide causes a significant reduction in mortality rate in cell lines, which are referred as dose dependent. Hence, in comparison to Caco-2 and Hep-G2, the least concentration to alert the mortality and viability rate was for HT29, 40 µg/mL. It is noteworthy that the cytotoxic effect was remarkably higher on HT29 than Caco-2 and Hep-G2 with lower IC50 (107 µg/mL).
Apoptosis, a physiological mechanism of cellular demise, is prompted by intra- and extracellular signals. This process serves a crucial role in maintaining the balance and proper functioning of normal tissues during development and homeostasis. In the context of cancer progression, apoptosis acts as a barricade against the uncontrolled growth of transformed cells. Nevertheless, in tumors that have undergone significant transformation and exhibit resistance to therapeutic interventions, the occurrence of apoptosis may be reduced (6). Bcl2 and APAF1 are key factors in the process of cell death, which were evaluated by both MTT assay and real-time PCR; the latter is more sensitive and specific. In fact, releasing cytochrome c from mitochondria is the pivotal triggering episode associating a cell to apoptosis, resulting in the formation of apoptosome-containing caspases. Bcl2 as an antiapoptotic protein interferes in the permeabilization of the mitochondrial outer membrane, thus barricading apoptosis (24,25). Controversy, oligomerization of APAF1 in response to discharge of cytochrome c facilitates the formation of apoptosome, leading to downregulation of caspases and triggering apoptosis (26). In our study, there is an increase in gene expression level of Bcl2 gene but it wasn’t significant in Caco-2 cell line. A significant decrease in expression levels of Bcl2 and APAF1 genes was seen in both Hep-G2 and HT29 cell lines. On the basis of update by the Nomenclature Committee on Cell Death (NCCD), regulated cell death could be classified, based on its molecular characteristics, into multiple categories in which some of them were well studied like: apoptosis, necrosis, necroptosis, and pyroptosis, while others like ferroptosis, entotic, autophagy, etc., are underestimated and less well-studied (27). For this case, we could conclude that downregulation in any of the aforementioned gene expression levels may result in consequences of any of the aforesaid cell death types. D’Arcy states that the cell in media could react in different ways when it fails to maintain homeostasis with its environment. Hence, the remains of late apoptotic cells that have lost their integrity are simply described as necrosis (28).
For future prospect, conducting experiments on animal models and assessing cell death molecular hallmarks using blotting techniques would give a better point of view for conducting upcoming researches. Challenges include being unable to synthesize the peptide in our country and the need for implementing more accurate and specific tests for applying blotting tests and to evaluate whether the peptide could enter the cells.
Conclusion
The results demonstrated that T. gondii peptide exerted an influence on the mortality of cancer cells and altered the expression of apoptosis-related genes. However, further optimization and redesign of the peptide could enhance its potential as a cancer therapy. Given the potential of peptides as selective and potent therapeutic agents, enhancing them with nanoparticles and improving delivery systems could optimize their anticancer efficacy. Future research could focus on animal models and molecular analyses with blotting techniques to further evaluate and improve this promising therapeutic approach.
Disclosures
Authors’ contributions: All authors contributed to the study conception and design. Material preparation, data collection and analysis were performed by Javid Sadraei, Firooz Shahrivar, Majid Pirestani, and Ehsan Ahmadpour. The first draft of the manuscript was written by Firooz Shahrivar and all authors commented on previous versions of the manuscript. All authors read and approved the final manuscript.
Data availability declaration: The authors declare that data would be available if any formal request is sent to the corresponding author.
Funding: This study was funded by Tarbiat Modares University, Tehran, Iran (MED 87201).
Conflict of interest: The authors declare that they have no conflict of interest.
Ethical approval: This study was performed in line with the principles of the Declaration of Helsinki. Approval was granted by the Ethics Committee of Tarbiat Modares University (IR.MODARES.REC.1400.128).
Consent to publish/participate: The authors affirm that no patient/participant consent was required for publishing; meanwhile, there is no conflict or opposition for publishing the data.
References
- 1. Flegr J, Prandota J, Sovičková M, Israili ZH. Toxoplasmosis – a global threat. Correlation of latent toxoplasmosis with specific disease burden in a set of 88 countries. PLoS One. 2014;9(3):e90203. CrossRef PubMed
- 2. Dubey JP. History of the discovery of the life cycle of Toxoplasma gondii. Int J Parasitol. 2009;39(8):877-882. CrossRef PubMed
- 3. Attias M, Teixeira DE, Benchimol M, Vommaro RC, Crepaldi PH, De Souza W. The life-cycle of Toxoplasma gondii reviewed using animations. Parasit Vectors. 2020;13(1):588. CrossRef PubMed
- 4. Stelzer S, Basso W, Benavides Silván J, et al. Toxoplasma gondii infection and toxoplasmosis in farm animals: risk factors and economic impact. Food Waterborne Parasitol. 2019;15:e00037. CrossRef PubMed
- 5. Dubey JP. The history and life cycle of Toxoplasma gondii. In: Weiss LM, Kim K, eds. Toxoplasma gondii. Elsevier 2020; 1-19. CrossRef
- 6. Hanahan D, Weinberg RA. Hallmarks of cancer: the next generation. Cell. 2011 Mar 4;144(5):646-74. CrossRef PubMed
- 7. Callejas BE, Martínez-Saucedo D, Terrazas LI. Parasites as negative regulators of cancer. Biosci Rep. 2018;38(5):BSR20180935. CrossRef PubMed
- 8. Sung H, Ferlay J, Siegel RL, et al. Global cancer statistics 2020: GLOBOCAN estimates of incidence and mortality worldwide for 36 cancers in 185 countries. CA Cancer J Clin. 2021;71(3):209-249. CrossRef PubMed
- 9. Knoll LJ, Hogan DA, Leong JM, Heitman J, Condit RC. Pearls collections: What we can learn about infectious disease and cancer. Public Library of Science San Francisco 2018; e1006915.
- 10. Bahadory S, Sadraei J, Zibaei M, Pirestani M, Dalimi A. In vitro anti-gastrointestinal cancer activity of Toxocara canis-derived peptide: analyzing the expression level of factors related to cell proliferation and tumor growth. Front Pharmacol. 2022;13:878724. CrossRef PubMed
- 11. Fox BA, Butler KL, Guevara RB, Bzik DJ. Cancer therapy in a microbial bottle: uncorking the novel biology of the protozoan Toxoplasma gondii. PLoS Pathog. 2017;13(9):e1006523. CrossRef PubMed
- 12. Soleimani M, Nadri S. A protocol for isolation and culture of mesenchymal stem cells from mouse bone marrow. Nat Protoc. 2009;4(1):102-106. CrossRef PubMed
- 13. Kumar P, Nagarajan A, Uchil PD. Analysis of cell viability by the MTT assay. Cold Spring Harb Protoc. 2018;2018(6):pdb.prot095505. CrossRef PubMed
- 14. Pan W, Yang J, Wei J, et al. Functional BCL-2 regulatory genetic variants contribute to susceptibility of esophageal squamous cell carcinoma. Sci Rep. 2015;5(1):11833. CrossRef PubMed
- 15. Shang J, Yang F, Wang Y, et al. MicroRNA-23a antisense enhances 5-fluorouracil chemosensitivity through APAF-1/caspase-9 apoptotic pathway in colorectal cancer cells. J Cell Biochem. 2014;115(4):772-784. CrossRef PubMed
- 16. McKinnon KM. Flow cytometry: an overview. Curr Protoc Immunol. 2018;120(1):5.1.1-5.1.11. CrossRef
- 17. Lin SR, Chang CH, Hsu CF, et al. Natural compounds as potential adjuvants to cancer therapy: preclinical evidence. Br J Pharmacol. 2020;177(6):1409-1423. CrossRef PubMed
- 18. Zheng H-C. The molecular mechanisms of chemoresistance in cancers. Oncotarget. 2017;8(35):59950-59964. CrossRef PubMed
- 19. Chen M-L, Lai C-J, Lin Y-N, Huang C-M, Lin Y-H. Multifunctional nanoparticles for targeting the tumor microenvironment to improve synergistic drug combinations and cancer treatment effects. J Mater Chem B. 2020;8(45):10416-10427. CrossRef PubMed
- 20. Garcia SB, Aranha AL, Garcia FRB, et al. A retrospective study of histopathological findings in 894 cases of megacolon: what is the relationship between megacolon and colonic cancer? Rev Inst Med Trop São Paulo. 2003;45(2):91-93. CrossRef PubMed
- 21. Hunter CA, Subauste CS, Van Cleave VH, Remington JS. Production of gamma interferon by natural killer cells from Toxoplasma gondii-infected SCID mice: regulation by interleukin-10, interleukin-12, and tumor necrosis factor alpha. Infect Immun. 1994;62(7):2818-2824. CrossRef PubMed
- 22. Fosgerau K, Hoffmann T. Peptide therapeutics: current status and future directions. Drug Discov Today. 2015;20(1):122-128. CrossRef PubMed
- 23. Lv S, Sylvestre M, Prossnitz AN, Yang LF, Pun SH. Design of polymeric carriers for intracellular peptide delivery in oncology applications. Chem Rev. 2021;121(18):11653-11698. CrossRef PubMed
- 24. Gogvadze V, Orrenius S, Zhivotovsky B. Multiple pathways of cytochrome c release from mitochondria in apoptosis. Biochim Biophys Acta. 2006;1757(5-6):639-647. CrossRef PubMed
- 25. Brunelle JK, Letai A. Control of mitochondrial apoptosis by the Bcl-2 family. J Cell Sci. 2009;122(Pt 4):437-441. CrossRef PubMed
- 26. Shakeri R, Kheirollahi A, Davoodi J. Apaf-1: regulation and function in cell death. Biochimie. 2017;135:111-125. CrossRef PubMed
- 27. Tang D, Kang R, Berghe TV, Vandenabeele P, Kroemer G. The molecular machinery of regulated cell death. Cell Res. 2019;29(5):347-364. CrossRef PubMed
- 28. D’Arcy MS. Cell death: a review of the major forms of apoptosis, necrosis and autophagy. Cell Biol Int. 2019;43(6):582-592. CrossRef PubMed
- 29. Robles AI, Bemmels NA, Foraker AB, Harris CC. APAF-1 is a transcriptional target of p53 in DNA damage-induced apoptosis. Cancer research. 2001 Sep 15;61(18):6660-4.
- 30. Liu L, Yuan G, Cheng Z, Zhang G, Liu X, Zhang H. Identification of the mRNA expression status of the dopamine D2 receptor and dopamine transporter in peripheral blood lymphocytes of schizophrenia patients. PLoS One. 2013;8(9):e75259. CrossRef PubMed