![]() |
Drug Target Insights 2024; 18: 84-93 ISSN 1177-3928 | DOI: 10.33393/dti.2024.3169 ORIGINAL RESEARCH ARTICLE |
![]() |
Cytotoxic activity, selectivity, and clonogenicity of fruits and resins of Saudi medicinal plants against human liver adenocarcinoma
ABSTRACT
Background: Edible fruits and resins provide various benefits to mankind including potential medicinal applications. This study aimed to determine the cytotoxicity, selectivity, and clonogenicity of fruits and exudates of certain Saudi medicinal plants (Anethum graveolens (BEP-09), Opuntia ficus-indica (L.) Miller (BEP-10), Boswellia serrata Roxb. ex Colebr. (BEP-11), and Commiphora myrrha (BEP-12)) against human liver adenocarcinoma (HepG2).
Methods: Initial cytotoxicity and cell line selectivity against different cell lines were screened using MTT assay. The most promising extract was subjected to gas chromatography-mass spectrometry (GC-MS) analysis to determine the main phytoconstituents. Clonogenicity was checked for the most active extract.
Results: The selected plants’ fruits and resins possess a significant cytotoxic activity estimated as IC50. The fruit of BEP-10 was found to be the most active extract against liver cancer cells (IC50 = 2.82) comparable to both doxorubicin (IC50 = 1.40) and camptothecin (IC50 = 1.11). It showed a selectivity index of 4.47 compared to the normal human foetal lung fibroblast (MRC5) cells. BEP-10 showed a dose-dependent clonogenic effect against HepG2 cells comparable to the effect of doxorubicin. The GC-MS chromatogram of BEP-10 extract revealed the presence of eight small polar molecules, representing 73% of the total identified compounds and the rest three molecules (27%) were non-polar constituents. The furan derivatives represent the chief components in BEP-10 (16.3%), while the aldehyde 5-(hydroxymethyl)-2-furancarboxaldehyde was found to be the main molecule (13.2%).
Conclusion: The fruits of BEP-10 have a potential cytotoxic effect particularly against HepG2. The identified phytoconstituents in the tested plant extract might contribute to the investigated cytotoxic activity.
Keywords: Clonogenicity, Cytotoxicity, MTT assay, Opuntia ficus-indica, Saudi plants, Selectivity index
Received: June 16, 2024
Accepted: September 18, 2024
Published online: October 22, 2024
Corresponding author:
Ali Hendi Alghamdi
email: drahendi2030@gmail.com
Drug Target Insights - ISSN 1177-3928 - www.aboutscience.eu/dti
© 2024 The Authors. This article is published by AboutScience and licensed under Creative Commons Attribution-NonCommercial 4.0 International (CC BY-NC 4.0).
Commercial use is not permitted and is subject to Publisher’s permissions. Full information is available at www.aboutscience.eu
Introduction
Anethum graveolens L. is a member of the Apiaceae family locally known as Shabat-sanout. This plant has a long history of use as a spice in our food, where its seeds and leaves are used as flavouring agents. It is an erect, robust, and rather glabrous annual aromatic herb. The leaves are three to four pinnate, with the ultimate segments narrowly linear to filiform. The flowers are yellow, and appear in umbels, with an elliptic cremocarp. It has been recognized in different systems of traditional medicine for the treatment of different diseases and ailments of humans. The plant is used as an antispasmodic, carminative, and anti-inflammatory. It is also used as medicine for loss of appetite, cough and cold, menstrual cramps, liver problems, oral care, strengthening the immune system, protection against bone degradation, and urinary tract disorders (1). The antioxidant and anticancer activities of A. graveolens were investigated in human, lung, breast, and cervical carcinoma cell lines (2-4). Nam et al (5) studied the anti-inflammatory and protective properties of A. graveolens (dill seeds) on oesophageal mucosal damage in rats induced by reflux esophagitis and revealed good physiological activity and the possibility of being used as a medicinal, food, and functional resource for the prevention and therapy of gastro-oesophageal disorders. A systematic review and meta-analysis of randomized controlled trials investigated the effects of A. graveolens (dill) supplementation on lipid profile and glycaemic control, showing that A. graveolens could provide favourable effects on insulin resistance and serum low-density lipoprotein (6). The anthelmintic action of A. graveolens essential oil was found to be a promising alternative in the control of sheep gastrointestinal nematodes (7). Khare (8) reported that it was used for eye problems.
Opuntia ficus-indica (L.) Miller is a member of the family of Cactaceae locally known as ElBarshoumy–El TeenElShawki. It is a shrub or arborescent. Leaves are subulate and deciduous. Fruits are ellipsoidal or obovoid, red, yellow to orange, fleshy, edible. The plant is widely distributed in the south and the southwest of Saudi Arabia. It is widely known for its beneficial properties (9). Historically it was used as food for humans and farm animals and in folk medicine due to its nutritional properties and beneficial activities (10). Traditional medicine has used many plant extracts for human and animal wellness, due to their beneficial properties in wound healing and skin. In this regard, the study of Trombetta et al (11) is most helpful. Traditionally it was used as a treatment for gastritis, hyperglycaemia, hypercholesterolaemia, arteriosclerosis, diabetes, and prostatic hypertrophy, and it also has hypolipidaemic action and immune regulation function in the gastrointestinal tract (12). The protective properties of various plant extracts on airway inflammation related to exposure to PM10 and diesel exhaust particles were evaluated in mice (13). The antioxidants of O. ficus-indica as important inhibitors of free radical formation were reported by Castañeda-Arriaga et al (14), as well as antioxidants and inhibition of the sugar digestive enzyme activities of polyphenols by in vitro experiments (15). The powder of peel and seed of the plant efficiently removes the aqueous manganese cations (16). The gums were used to improve the quality of breads and cakes (17). The phenolic phytoconstituents, antioxidant and antiacetylcholinesterase activities of O. ficus-indica peel and flower teas were evaluated after in vitro gastrointestinal digestion (18). It modulates the intestinal microbiome in obese women and improves host metabolism (19). Polysaccharides from O. ficus-indica showed a regulating effect on intestinal flora of cyclophosphamide-induced immunosuppressed mice by effectively increasing the white blood cell count index and improving their thymus and spleen, while effectively promoting the secretion of interleukin (IL)-4, IL-1beta, tumour necrosis factor (TNF)-alpha and interferon (IFN)-gamma (20). Indicaxanthin isolated from fruits enhances glucose dysmetabolism and reduces insulin resistance in mice fed the high-fat diet (21).
Boswellia serrata Roxb. ex Colebr. belongs to the Burseraceae family locally known as luban-Kundur. These are moderate to large deciduous trees. They have papery flakes of bark and “yellowish green resin” inside. Leaves are compound and alternate. Flowers are white and are distributed in southern Saudi Arabia.
The extract of B. serrata exhibited a potential effect in protecting the intestinal epithelium compared to lipopolysaccharide (LPS)-stimulated cells (22,23). The diuretic activity of gum extract in albino rats was investigated (24,25) and significant diuretic, kaliuretic, and natriuretic effects were observed. Synergistic antimicrobial activity of essential oil from B. serrata was studied with various azoles against azole-resistant strains of Candida albicans pathogens (26). The plant was used as a culture medium for micropropagation and as a natural source of nonsteroidal anti-inflammatory and antiarthritic agents (27). The antianaphylactic and mast cell stabilizing effects of boswellic acid have been assessed on passive paw anaphylaxis and revealed potential immunomodulatory activity (28). Recently, Boswellia spp. and its isolated bioactive phytoconstituents were traditionally used to treat chronic disease, inflammation, oral health, and microbial infection (29). Gum is traditionally used for the treatment of various inflammations that affect the skin, gums, eye, gastrointestinal tract (GIT) in addition to respiratory inflammation disorders such as bronchitis, asthma, laryngitis, etc. (30).
Commiphora myrrha (Nees) Engl. belongs to the Burseraceae family, locally known as El Murr Elihejazi. These are spiny, deciduous, almost shrub or small tree, with short thorns, producing a hard translucent yellowish gum resin. Leaves are green to greyish or glaucous, variable in shape, and minute in size. Native to Saudi Arabia, the plant is traditionally used as an anti-inflammatory and in the treatment of infectious diseases, making it a very popular and valuable alternative and traditional medicine (31,32). It was found to heal wounds, ulcers, and various diseases of the pulmonary, GIT, and urinary system (33).
Furanodienone and curzerene are bioactive components detected in the oil of the resinous exudate of C. myrrha that were tested and found to influence the spread of viruses by intervening at different stages of the virus life cycle (34). The antiosteoporotic effects of C. myrrha and its polysaccharide were inhibited through osteoclastogenesis (35). Sesquiterpenoids and its phytoconstituents isolated from the resinous exudate of C. myrrha were found to inhibit the migration of human hepatocellular liver carcinoma cells (HepG2) according to a dose-dependent pattern (36). A pilot study revealed that C. myrrha has significant analgesic properties (37). A combination of herbs (Commiphora mukul, C. myrrha, and Terminalia chebula) functions as an antioxidant, hypolipidemic, and antidiabetic substance; it could be recommended as a helpful herbal remedy for those with diabetes (38). The ethanolic extract of the resin of C. myrrha showed anti-obesity potential (39). It showed a hepatoprotective effect against D-GalN/LPS-induced liver injury in a rat model through multiple pathways (40). Murr (C. myrrha) is beneficial in treating eye diseases, as kahl forms in ulcers of the eye with other drugs. In Unani medicine, Murr is applied as a mixture with aabe mooli (radish juice) to eyes for cataracts, where the eyes are cleaned after dissolving murr in milk and in infraorbital haemorrhage (41-44).
Tumour-related destructive autoimmune responses can affect the eye, where autoantibody-mediated destruction of retinal cells is induced by ectopic expression of peripheral tumour-related ocular antigens (45). Neuroendocrine tumours can metastasize to the orbits of the eyes of the midgut carcinoid (46). In the Philippines, the majority of conjunctival, eyelid, and orbit tumours were benign, and retinoblastoma was the most prevalent type of intraocular tumour, while the majority of them were malignant (47). An update is needed to reorient the way to predict the prognosis of paediatric cancers, such as rhabdomyosarcoma and retinoblastoma, and also adult cancers, such as uveal melanoma and lymphomas, and the benefit of targeted therapies, immunotherapy, or even chemotherapy (48).
Fruits and resins are usually used as nutritional supplements and are rarely used for medical purposes. Attempts are being made to look for the constituents of the plant that can prevent and reverse cancer. In this study, in vitro anticancer activity and cell line selectivity of two fruits and two resins were studied in three different cell lines, while clonogenicity was investigated against HepG2. Furthermore, the most promising extract was subjected to gas chromatography-mass spectrometry (GC-MS) analysis to determine the main active phytoconstituent(s).
Materials and methods
Phytochemical studies
Identification of plant materials
Four plants – A. graveolens (fruit, coded as BEP-09), O. ficus-indica (fruit, BEP-10), B. serrata (resin, BEP-11), and C. myrrha (resin, BEP-12) (Fig. 1) – were identified and taxonomically classified by an expert taxonomist (Dr. Mohamed, HAA, Department of Biology, Faculty of Sciences, Al-Baha University) and were compared to herbarium materials and different volumes of the flora of Saudi Arabia (49-51). Voucher herbarium specimen numbers (BUH-76,77,78, and 79) were deposited at the Department of Biology of the Faculty of Science of Al-Baha University.
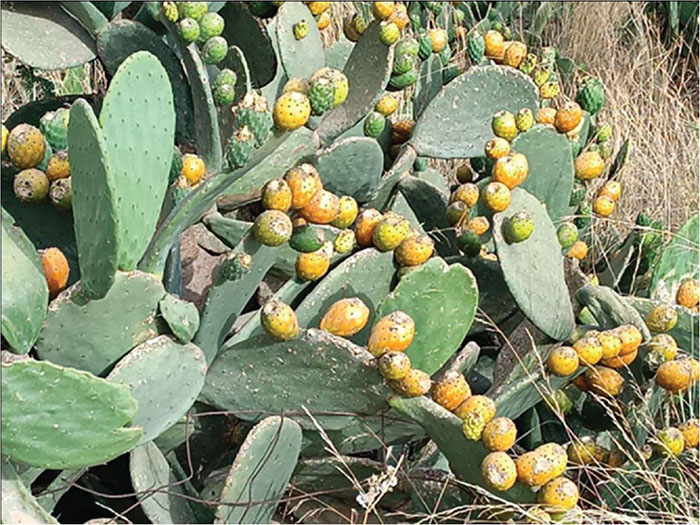
FIGURE 1 - Opuntia ficus-indica (L.) Miller (BEP-10) grows in the Al-Baha area, KSA.
Collection and extraction of plant materials
Plant specimens were collected from different sites in Baljurashi province (Wadi El khaitan), Al-Baha area, in April 2021. Fruits (1 kg) and resins (1 kg) were shade-dried and then powdered using a mechanical grinder. The dried materials were macerated in 80% ethanol v/v) for 1 week at room temperature. The resulting residues were filtered, pooled, and evaporated to dryness to provide viscous green to brownish syrups. The crude extracts, so obtained, were transferred to a Petri plate, allowed to dry, and finally weighed.
The percentage of yield was calculated using the formula: yield% = (Afforded extract weight)/(Air-dried weight) × 100.
The plants yielded extracts weighing 1.53, 1.73, 1.62, and 2.17 g, respectively.
GC-MS analysis
The dried fruits of O. ficus-indica (L.) Miller (BEP-10) were dissolved in methanol to reach a concentration of 1 mg/mL and diluted 1:10 v/v in methanol (100 μg/mL). The diluted sample was analysed using a GC-MS instrument (Thermo Scientific, USA) attached to a trace ultra-GC and ISQ detector and an AS 3000 autosampler. The separation of components was carried out using a TR-5MS column (Thermo Scientific, USA) with a length of 30 cm, a diameter of 0.25 mm, and a film thickness of 0.25 mm. Helium was used as a carrier gas at 1.2 mL/min with constant flow. The injection port was set at 32°C for 5 minutes, followed by a ramp to 205°C at a rate of 5°C/min and a hold time of 5 minutes. This was followed by a ramp to 280°C at a rate of 5°C/min and hold time of 5 minutes and at the end to 300°C at a rate of 5°C/min and a hold time of 5 minutes. The maximum oven temperature was set at 320°C. A volume of 2 μL diluted extract was injected into the system in split mode with the mass spectrometer run in electron ionization mode with 0.6 scan periods throughout the mass range of 60-900 amu (minutes). Both the temperature of the MS ion source and the transfer line were adjusted to 320°C and 350°C, respectively, using a 1 kV electron multiplier voltage.
Identification of phytoconstituents
Xcalibur software was used for mass spectral data analysis and the fragmentation patterns of each constituent were matched with MS data in the instrument database and built-in libraries including MAINLIB, NIST, and REPLIB. The phytocompounds present in the extract were identified by comparing them with the structures available in the computer library, and the percent abundance of each component was determined using the peak area as reference. The reported biological properties of the detected compounds are based on data from Duke’s Phytochemical and Ethnobotanical Database (52).
Cancer cell studies
Cancer cell culture
In this study, three cancer cell lines, MCF7 (human breast adenocarcinoma), HT29 (human colorectal adenocarcinoma), and HepG2 (human liver adenocarcinoma), were used, in addition to MRC5 (normal human foetal lung fibroblast), all were from American Type Culture Collection (ATCC), USA. Three cancer cells were subcultured in RPMI-1640 medium (10% foetal bovine serum (FBS)), while MRC5 was preserved in Eagle’s Minimum Essential Medium (EMEM, 10% FBS) – all at 37°C, 5% CO2, and 100% relative humidity, for a maximum of 5-10 passages.
Cytotoxicity and selectivity studies
The cytotoxic effect of four extracts, in addition to doxorubicin and camptothecin, was evaluated by the MTT assay, as reported by Alsanosy et al (53) and Abdalla et al (54). Each cell line was cultured separately in 96 wells (3 ×103/well) and incubated with each of the extracts or doxorubicin at a final concentration of 0-100 μg/mL, for 3 days at 37°C overnight (dimethyl sulfoxide (DMSO) 0.1%; n = 3 of three independent experiments). After 3 days of incubation, the cytotoxicity of each extract was evaluated using an MTT assay. MTT was added to each well in culture medium at a concentration of 0.5 mg/mL and incubated for 3 hours at 37°C. The MTT solution was removed and the formazan granules were dissolved by DMSO. The absorbance was read on a multiplate reader (BIORAD, PR 4100, Hercules, CA, USA). The optical density of the purple formazan A550 is proportional to the number of viable cells. The extract concentration causing 50% inhibition (IC50), compared to the control group, 100% cell growth, was estimated using GraphPad Prism. The selectivity index (SI) for the five extracts was calculated by dividing its IC50 for MRC5 cells by the IC50 for MCF7, HT29, or HepG2 cells.
Clonogenic assay
The clonogenic assay measures tumour cell survival and subsequent proliferative ability after drug exposure (55). The extract (BEP-10) was selected for a further clonogenic test, as it showed the highest selectivity to the normal cell line MRC5. Exponentially growing HepG2 cells in DMEM (supplemented with 10% FBS and 1% penicillin/streptomycin) were seeded in duplicates at a density of 200 cells/well in a 6-well plate and allowed to attach overnight and then exposed to an increasing concentration of BEP-10 (0, 0.75, 1.5, 2.25 µg/mL) for 72 hours. The wells containing the extract were then replaced with fresh media without the extract. The cells were left to grow at 37°C, 5% CO2, and 100% humidity. Daily wells were checked and the cells that form colonies were roughly counted. After 14 days, plates were rinsed in phosphate-buffered saline and fixed with pre-chilled methanol at room temperature for 20 minutes, then stained with 0.5 methylene blue in 1:1 methanol/H2O (v/v) for 10 minutes, washed thoroughly in dH2O, and air dried. Cell colonies were counted and recorded macroscopically.
Ethics approval of the study
According to the standards of Al-Baha University, all funded project proposals have undergone a critical review followed by approval by relevant scientific research committees before acceptance.
Results
Phytochemical studies
The four plant extracts produced the following yields: BEP-09 (15.3%), BEP-10 (17.3%), BEP-11 (16.2%), and BEP-13 (21.7) from fruits and resins (Fig. 2).
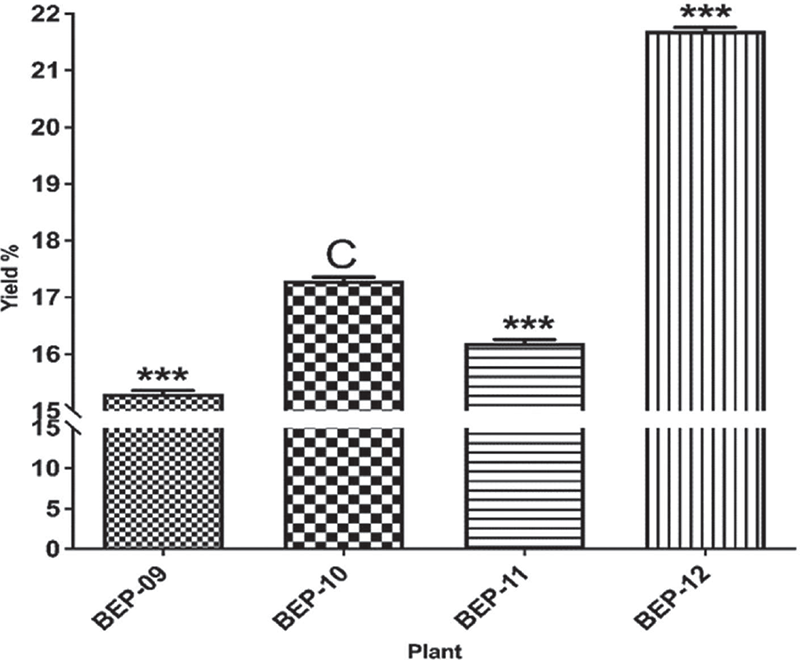
FIGURE 2 - Yield % of dry extracts obtained after ethanolic extraction and evaporation of four different selected plants. C; control, ***; p ≤ 0.001.
Identification of phytoconstituents using GC-MS
Investigation of the GC-MS chromatogram (see the supplementary file) of the fruits of the Miller plant O. ficus-indica (L.) (BEP-10 extract) indicated the presence, mainly, of eight small polar molecules (18) (Tab. 1 and Fig. 3). These molecules represent 73% of the total identified compounds. The rest (27%) were the non-polar constituents represented by compounds 9‒11. Therefore, polar molecules constitute >40% of the peak area % relative to the total peak area % of the components that existed in BEP-10 extract. In contrast, non-polar residues represented only 8% of the total peak area % of the components that existed in BEP-10 extract. 5-(Hydroxymethyl)-2-furancarboxaldehyde (1) was found to be the main molecule in BEP-10 extract (13.2%). The other furan derivative (8) was found to have a peak area % of 3.1. Thus, furan derivatives represent the main component of BEP-10 extract (Fig. 4) while phenolic derivatives represented by compounds 2, 3, and 6 came at the second level (peak area % = 10.05) with carbohydrates 4 and 5 (peak area % = 9.91).
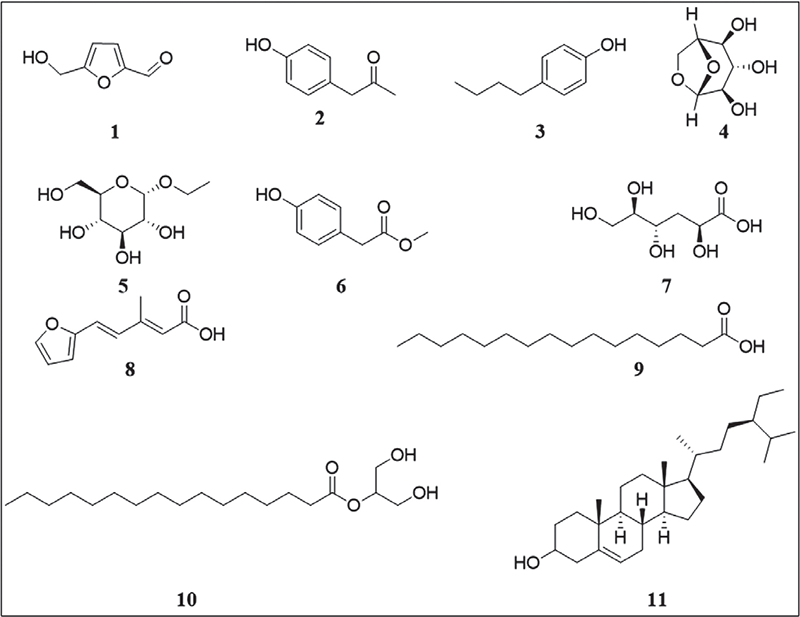
FIGURE 3 - Structures of chemical constituents identified by gas chromatography-mass spectrometry for the fruits of Opuntia ficus-indica (L.) Miller (BEP-10 extract).
Compound | Formula | Molecular weight | Peak area (%) | Retention time (minutes) | Biological activity |
---|---|---|---|---|---|
(1) 5-(hydroxymethyl)-2-furancarboxaldehyde |
C6H6O3 | 126.11 | 13.2 | 5.875 | Known to be associated with antimicrobial properties (56) used as an antifungal (57) |
(2) 1-(4′-Hydroxyphenyl)-2-propanone |
C9H10O2 | 150.17 | 2.52 | 7.752 | Exhibits a myriad of pharmacological actions, such as antimicrobial, antitussive, antispasmodic, and anticancer properties (58) |
(3) 4-Butyl-phenol |
C10H14O | 150.22 | 2.93 | 8.011 | No significant report |
(4) 1,6-Anhydro-beta-D-glucopyranose |
C6H10O5 | 162.14 | 5.31 | 8.328 | No significant report |
(5) Ethylalpha-d-glucopyranoside |
C8H16O6 | 208.09 | 4.60 | 9.245 | Maintenance and improvement of skin homeostasis and moisturizing functions (59) |
(6) Benzeneacetic acid, 4-hydroxy-, methyl ester |
C9H10O3 | 166.06 | 4.66 | 9.438 | - |
(7) 3-Deoxy-d-mannonic acid |
C6H12O6 | 180.16 | 6.43 | 9.651 | - |
(8) 5-(2-Furyl)-3-methyl-penta-2,4-dienoic acid |
C10H10O3 | 178.18 | 3.1 | 9.843 | - |
(9) n-Hydroxydecanoic acid |
C16H32O2 | 256.42 | 1.89 | 11.347 | As anti-inflammatory (60), cytotoxic activity (61) |
(10) Hexadecanoic acid, 2-hydroxy-1-(hydroxymethyl)ethyl ester |
C19H38O4 | 330.50 | 3.01 | 14.656 | - |
(11) Stigmast-5-en-3-ol |
C29H50O | 414.71 | 1.54 | 23.313 | Apoptotic and antiproliferative effects (62) |
GC-MS = gas chromatography-mass spectrometry.
Cytotoxicity and cell line selectivity studies
The four extracts showed a variable IC50 ranging from 0.75 to 19.32 μg/mL. The most active extract was BEP-10 against HepG2 cells, and showed ~4.5-fold selectivity compared to normal MRC5 cells. The selectivity of the extract BEP-10 was greater than that of doxorubicin and camptothecin (Tabs. 2 and 3).
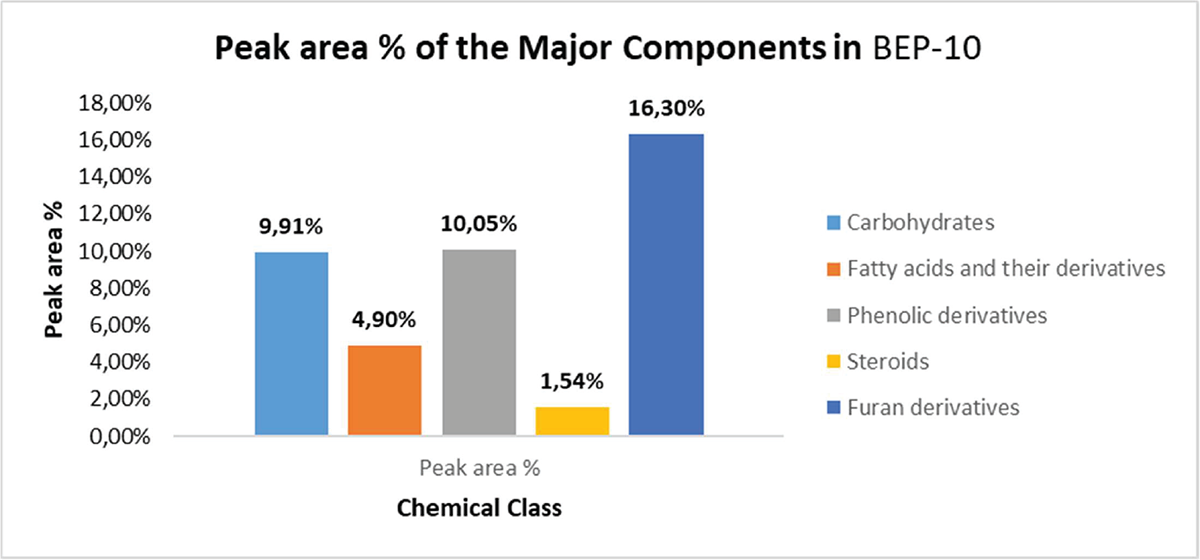
FIGURE 4 - Peak areas (%) for the major components of Opuntia ficus-indica (L.) Miller (BEP-10 extract).
Extract | MCF7 | HT29 | HepG2 | Average* IC50 | MRC5 |
---|---|---|---|---|---|
BEP-09 | 6.00±1.61 | 8.20±0.57 | 5.20±0.58 | 6.47 | 2.94±0.71 |
BEP-10 | 1.85±0.73 | 5.85±0.23 | 0.75±0.11** | 2.82** | 3.34±0.41 |
BEP-11 | 16.93±0.66 | 16.07±0.11 | 14.07±1.04 | 15.69 | 10.77±0.54 |
BEP-12 | 16.53±0.43 | 19.32±0.64 | 9.60±0.82 | 15.15 | 8.72±1.56 |
Doxorubicin | 0.07±0.01 | 1.98±0.10 | 2.15±0.15 | 1.40 | 5.86±0.35 |
Camptothecin | 0.08±0.01 | 2.50±0.26 | 0.76±0.07 | 1.11 | 1.18±0.10 |
*Average cytotoxicity (IC50) of each extract against the three cancer cells. **p ≤ 0.01.
Extract | MRC5 | HT29 | HepG2 |
---|---|---|---|
BEP-09 | 0.49 | 0.36 | 0.57 |
BEP-10 | 1.80 | 0.57 | 4.47 |
BEP-11 | 0.64 | 0.67 | 0.77 |
BEP-12 | 0.53 | 0.45 | 0.91 |
Doxorubicin | 78.57 | 2.96 | 2.73 |
Camptothecin | 13.80 | 0.47 | 1.55 |
Clonogenic effect of the extract BEP-10 against HepG2 cells
The extract BEP-10 was tested for its possible clonogenic effect against HepG2 liver cancer cells. The extract revealed a dose-dependent clonogenic activity against a dose-dependent effect against HepG2 cells that was comparable to the effect of doxorubicin on the same cancer cells (Fig. 5).
Discussion
Fruits and resins have interesting medicinal uses. In vitro anticancer activity, cell line selectivity, and clonogenicity were considered as a useful trend to scavenge for a useful natural therapeutic agent(s) with putative anticancer property.
The extract of O. ficus-indica (fruits; BEP-10) yielded 17.3%. This indicates the high amounts of constituents that are expected to be available in these fruits.
The MTT assay of the four extracts showed a variable IC50 ranging from 0.75 to 19.32 μg/mL comparable to both standards: doxorubicin (IC50 = 1.40) and camptothecin (IC50 = 1.11), respectively. Fruits appear to be more effective than resins, because they showed lower IC50 values than those produced by standard drugs. These results were consistent with those of Castañeda-Arriaga et al (14) who studied the antioxidant effect of this plant and found that its chelating compounds can reduce the harmful effects caused by the most reactive free radical existing immediately.
The resulting selectivity (~4.5 fold) of the most active extract BEP-10 against HepG2 cells compared to normal MRC5 cells was found to be higher than that of doxorubicin and camptothecin. The extract BEP-10 was considered for more cytotoxic and mechanistic studies. Selectivity indicates the ability of the extract to have a maximum effect on cancerous cells and a lesser effect on normal cells. This indicates both its safety and efficacy, and thus it can serve as a promising and useful drug candidate (63).
Due to its high selectivity for HepG2 liver cancer cells, the extract BEP-10 was chosen to test its possible clonogenic effect and showed a dose-dependent clonogenic effect comparable to the effect of doxorubicin in the same cells. The macroscopically counted cell colonies indicate the suppression ability of the active extract, which can be taken as evidence to support the preliminary cytotoxicity and selectivity effects. A study by Terzo et al (21) revealed that the O. ficus-indica fruit extract exerted significant antioxidant and anti-inflammatory effects.
Correlating the cytotoxic activity of the most promising BEP-10 extract with its phytochemical constituents, GC-MS was performed and different classes of phytoconstituents were detected, including polar molecules (73%) and lipophilic constituents (27%). These have been reviewed as anti-inflammatory (64), antioxidant (65), and anticancer agents (66).
Our results showed that in compound 1, aldehyde 5-(hydroxymethyl)-2-furancarboxaldehyde, the furan derivative was the main compound of the BEP-10 extract. The literature revealed that the medicinal properties of furan include anticancer, antidepressant, antianxiolytic, analgesic, anti-inflammatory, muscle relaxant, antihypertensive, antiarrhythmic, antimicrobial like antibacterial, antifungal, or antiviral (67), anti-ageing agents, anti-ulcer, antihistaminic, anticholinergic, antiparkinsonian, antidiuretic, and inhibition of sickle cell formation (68).
However, the GC-MS chromatogram showed three phenolic derivatives (compounds 2, 3, and 6) that were classified as second contents in the BEP-10 extract. These findings were consistent with various studies such as the anticancer (69), anti-trypanosomal activity (70), antileishmanial, anti-inflammatory and antimicrobial activities (71), and anti-neuroinflammatory and neuroprotective activities (72).
Conclusion
The study concludes that the O. ficus-indica fruit (BEP-10) is widely distributed in the Al-Baha area and is locally considered a popular fruit. Its extract showed a significant cytotoxic effect, particularly against HepG2 liver cancer cells with high cell selectivity.
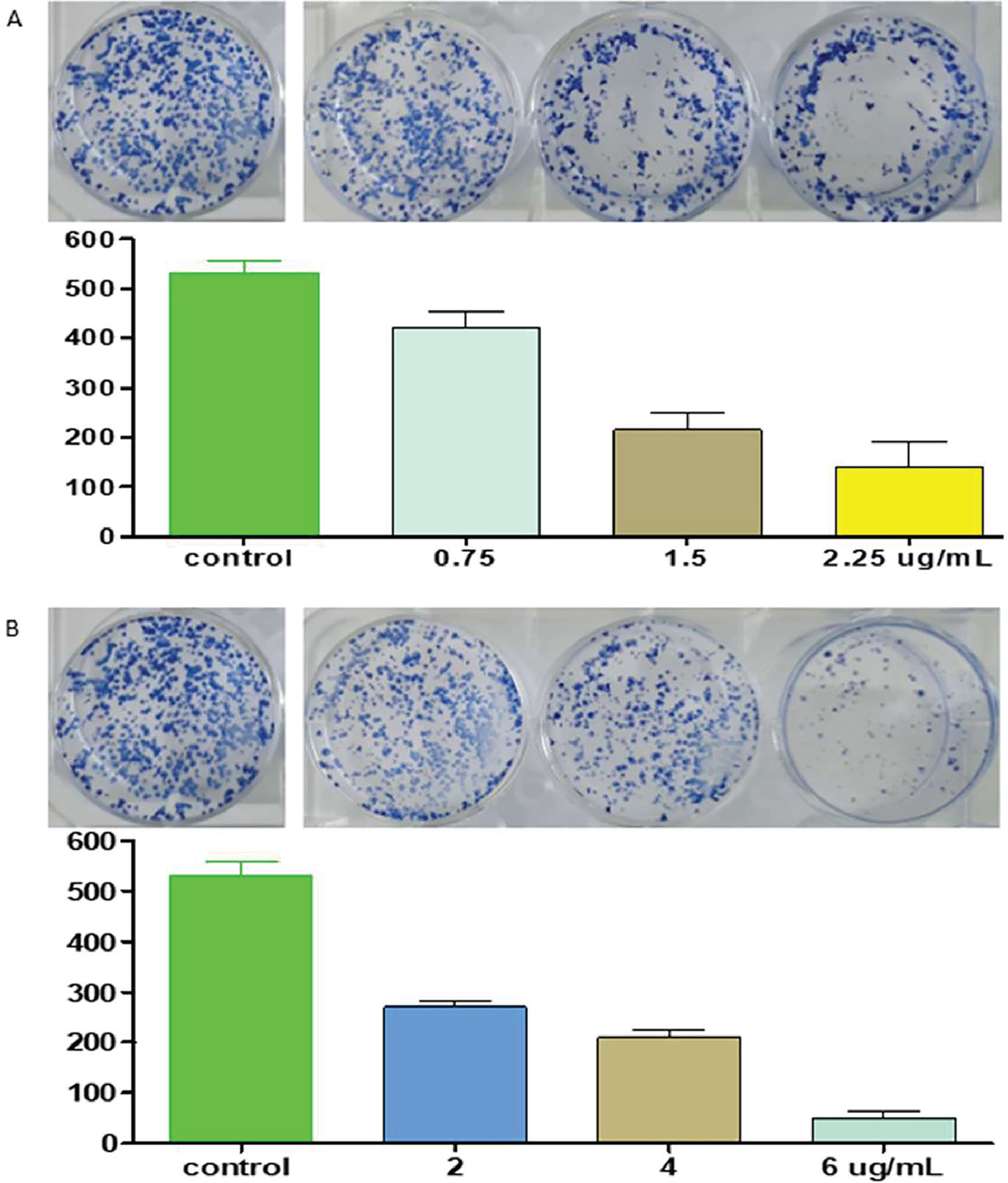
FIGURE 5 - Colonies of HepG2 cells treated with A) extract BEP-10 (0, 0.75, 1.5, 2.25 µg/mL; n = 2), and B) doxorubicin (0, 2, 4, and 6 µg/mL; n = 2) for 72 hours in 6-well plates followed by a 14-day period of incubation without extract. Bar graph showing x-axis: extract concentrations (BEP-10) or doxorubicin concentrations; and y-axis: colony number. Results are expressed as cell number ± standard deviation of two independent experiments.
Polar and lipophilic phytoconstituents were identified in the plant extract and could contribute to the investigated cytotoxic activity. The furan derivatives that are present as the main compound may play a vital role in the activity studied. Further research is required to obtain the profile of the drug candidate.
Acknowledgements
This article is a part of the funded project: Investigation of Medicinal Plants with Putative Ocular Effects from Al-Baha Area, Southwestern, Saudi Arabia, Grant number (MOE-BU-4-2020); thus, the funder body is highly acknowledged for their financial support.
Disclosures
Data Availability Statement: All data generated or analysed during this study are available with Dr. Ali as the correspondence author and can be provided upon request.
Conflict of interest: The authors declare that they have no competing interests.
Funding: The author(s) disclosed receiving the following financial support for the research, authorship, and/or publication of this article. This work was supported by the Department of Research and Innovation of the Ministry of Education of Saudi Arabia (grant number: MOE-BU-4-2020). Sponsors did not play a role in the design of the study, the collection and analysis of data, or the preparation of the manuscript.
Authors’ contribution: Ali conceived the original idea. Mahadi, Hiadar, and Aimun designed the study. Ashraf, Mohamed EA, Riyad A, and Bassem R conducted the experimental work and collected the data. Aimun analysed the data and drafted the manuscript. Ali and Asaad, revised it. All approved the final version that was submitted. All authors equally contributed to the whole work preparation. All authors approved the version to be published and agreed to be accountable for all aspects of the work.
References
- 1. Meena SS, Lal G, Dubey PN, Meena MD. Medicinal and therapeutic uses of Dill (Anethum graveolens L.) – a review. International J Seed Spices. 2019;9(1):14-20. Online
- 2. Al-Oqail MM, Farshori NN. Antioxidant and anticancer efficacies of Anethum graveolens against human breast carcinoma cells through oxidative stress and caspase dependency. BioMed Res Int. 2021;2021:5535570. CrossRef PubMed
- 3. Kaur N, Chahal KK, Kumar A, Singh R, Bhardwaj U. Antioxidant activity of Anethum graveolens L. essential oil constituents and their chemical analogues. J Food Biochem. 2019;43(4):e12782. CrossRef PubMed
- 4. Al-Sheddi ES, Al-Zaid NA, Al-Oqail MM, Al-Massarani SM, El-Gamal AA, Farshori NN. Evaluation of cytotoxicity, cell cycle arrest and apoptosis induced by Anethum graveolens L. essential oil in human hepatocellular carcinoma cell line. Saudi Pharm J. 2019;27(7):1053-1060. CrossRef PubMed
- 5. Nam HH, Nan L, Choo BK. Anti-inflammation and protective effects of Anethum graveolens L. (Dill seeds) on esophageal mucosa damages in reflux esophagitis-induced rats. Foods. 2021;10(10):2500. CrossRef PubMed
- 6. Mousavi SM, Beatriz Pizarro A, Akhgarjand C, et al. The effects of Anethum graveolens (dill) supplementation on lipid profile and glycemic control: a systematic review and meta-analysis of randomized controlled trials. Crit Rev Food Sci Nutr. 2022;62(21):5705-5716 CrossRef PubMed
- 7. Castro LM, Pinto NB, Moura MQ, et al. Antihelminthic action of the Anethum graveolens essential oil on Haemonchus contortus eggs and larvae. Braz J Biol. 2021;81(1):183-188. CrossRef PubMed
- 8. Khare CP. Indian herbal remedies: rational Western therapy, ayurvedic and other traditional uses, botany. Springer 2004.
- 9. Aragona M, Lauriano ER, Pergolizzi S, Faggio C. Opuntia ficus-indica (L.) Miller as a source of bioactivity compounds for health and nutrition. Nat Prod Res. 2018;32(17):2037-2049. CrossRef PubMed
- 10. Alimi H, Hfaiedh N, Bouoni Z, et al. Antioxidant and antiulcerogenic activities of Opuntia ficus indica f. inermis root extract in rats. Phytomedicine. 2010 Dec 1;17(14):1120-6 CrossRef PubMed
- 11. Trombetta D, Puglia C, Perri D, et al. Effect of polysaccharides from Opuntia ficus-indica (L.) cladodes on the healing of dermal wounds in the rat. Phytomedicine. 2006;13(5):352-358. CrossRef PubMed
- 12. El-Mostafa K, El Kharrassi Y, Badreddine A, et al. Nopal cactus (Opuntia ficus-indica) as a source of bioactive compounds for nutrition, health and disease. Molecules. 2014;19(9):14879-14901. CrossRef PubMed
- 13. Lee YS, Yang WK, Park YR, et al. Opuntia ficus-indica alleviates particulate matter 10 plus diesel exhaust particles (PM10D)-induced airway inflammation by suppressing the expression of inflammatory cytokines and chemokines. Plants. 2022;11(4):520. CrossRef PubMed
- 14. Castañeda-Arriaga R, Perez-Gonzalez A, Marino T, Russo N, Galano A. Antioxidants into nopal (Opuntia ficus-indica), important inhibitors of free radicals’ formation. Antioxidants. 2021;10(12):2006. CrossRef PubMed
- 15. Zhou M, Wen C, Ming Y, Zhang L, Lyu X. [Distribution and bioactivity of polyphenols in Opuntia ficus-indica (L. ) Mill]. Wei Sheng Yan Jiu. 2022;51(3):463-469. PubMed
- 16. Djobbi B, Miled GLB, Raddadi H, Hassen RB. Efficient removal of aqueous manganese (II) cations by activated Opuntia ficus indica powder: adsorption performance and mechanism. Acta Chim Slov. 2021;68(3):548-561. CrossRef PubMed
- 17. Hussain S, Alamri MS, Mohamed AA, et al. Exploring the role of acacia (Acacia seyal) and cactus (Opuntia ficus-indica) gums on the dough performance and quality attributes of breads and cakes. Foods. 2022;11(9):1208. CrossRef PubMed
- 18. Amrane-Abider M, Nerín C, Tamendjari A, Serralheiro MLM. Phenolic composition, antioxidant and antiacetylcholinesterase activities of Opuntia ficus-indica peel and flower teas after in vitro gastrointestinal digestion. J Sci Food Agric. 2022;102(11):4401-4409. CrossRef PubMed
- 19. Corona-Cervantes K, Parra-Carriedo A, Hernández-Quiroz F, et al. Physical and dietary intervention with Opuntia ficus-indica (nopal) in women with obesity improves health condition through gut microbiota adjustment. Nutrients. 2022;14(5):1008. CrossRef PubMed
- 20. Liu Z, Zhang J, Zhao Q, Wen A, Li L, Zhang Y. The regulating effect of Tibet Opuntia ficus-indica (Linn.) Mill. polysaccharides on the intestinal flora of cyclophosphamide-induced immunocompromised mice. Int J Biol Macromol. 2022;207:570-579. CrossRef PubMed
- 21. Terzo S, Attanzio A, Calvi P, et al. Indicaxanthin from Opuntia ficus-indica fruit ameliorates glucose dysmetabolism and counteracts insulin resistance in high-fat-diet-fed mice. Antioxidants. 2021;11(1):80. CrossRef PubMed
- 22. Governa P, Marchi M, Cocetta V, et al. Effects of Boswellia Serrata Roxb. and Curcuma longa L. in an in vitro intestinal inflammation model using immune cells and CaCo-2. Pharmaceuticals (Basel). 2018;11(4):126. CrossRef PubMed
- 23. Catanzaro D, Rancan S, Orso G, et al. Boswellia serrata preserves intestinal epithelial barrier from oxidative and inflammatory damage. PLoS One. 2015;10(5):e0125375. CrossRef PubMed
- 24. Asif M, Jabeen Q, Abdul-Majid AM, Atif M. Diuretic activity of Boswellia serrata Roxb. oleo gum extract in albino rats. Pak J Pharm Sci. 2014;27(6):1811-1817. PubMed
- 25. Wahab, S.M.; Aboutabl, E.A.; El-Zalabani, S.M.; Fouad, H.A.; De Pooter, H.L.; El-Fallaha, B. The essential oil of olibanum. Planta Med. 1987, 53, 382–384. CrossRef PubMed
- 26. Sadhasivam S, Palanivel S, Ghosh S. Synergistic antimicrobial activity of Boswellia serrata Roxb. ex Colebr. (Burseraceae) essential oil with various azoles against pathogens associated with skin, scalp and nail infections. Lett Appl Microbiol. 2016;63(6):495-501. CrossRef PubMed
- 27. Nikam TD, Ghorpade RP, Nitnaware KM, Ahire ML, Lokhande VH, Chopra A. Micropropagation and non-steroidal anti-inflammatory and anti-arthritic agent boswellic acid production in callus cultures of Boswellia serrata Roxb. Physiol Mol Biol Plants. 2013;19(1):105-116. CrossRef PubMed
- 28. Pungle P, Banavalikar M, Suthar A, Biyani M, Mengi S. Immunomodulatory activity of boswellic acids of Boswellia serrata Roxb. Indian J Exp Biol. 2003;41(12):1460-1462. PubMed
- 29. Almeida-da-Silva CLC, Sivakumar N, Asadi H, et al. Effects of frankincense compounds on infection, inflammation, and oral health. Molecules. 2022;27(13):4174. CrossRef PubMed
- 30. Siddiqui MZ. Boswellia serrata, a potential antiinflammatory agent: an overview. Indian J Pharm Sci. 2011;73(3):255-261. PubMed
- 31. Shen T, Li GH, Wang XN, Lou HX. The genus Commiphora: a review of its traditional uses, phytochemistry and pharmacology. J Ethnopharmacol. 2012;142(2):319-330. CrossRef PubMed
- 32. Al-Robai SA, Ahmed AA, Mohamed HA, Ahmed AA, Zabin SA, Alghamdi AA. Qualitative and quantitative ethnobotanical survey in Al Baha province, southwestern Saudi Arabia. Diversity. 2022 Oct 13;14(10):867. CrossRef
- 33. Galehdari H, Negahdari S, Kesmati M, Rezaie A, Shariati G. Effect of the herbal mixture composed of Aloe Vera, Henna, Adiantum capillus-veneris, and Myrrha on wound healing in streptozotocin-induced diabetic rats. BMC Complement Altern Med. 2016;16(1):386. CrossRef PubMed
- 34. Madia VN, De Angelis M, De Vita D, et al. Oil and its main components for antiviral activity. Investigation of Commiphora myrrha (Nees) Engl. Pharmaceuticals (Basel). 2021;14(3):243. CrossRef PubMed
- 35. Hwang YH, Lee A, Kim T, Jang SA, Ha H. Anti-osteoporotic effects of Commiphora myrrha and its poly-saccharide via osteoclastogenesis inhibition. Plants. 2021;10(5):945. CrossRef PubMed
- 36. Ge CY, Zhang JL. Bioactive sesquiterpenoids and steroids from the resinous exudates of Commiphora myrrha. Nat Prod Res. 2019;33(3):309-315. CrossRef PubMed
- 37. Germano A, Occhipinti A, Barbero F, Maffei ME. A pilot study on bioactive constituents and analgesic effects of MyrLiq®, a Commiphora myrrha extract with a high furanodiene content. BioMed Res Int. 2017;2017:3804356. CrossRef PubMed
- 38. Sotoudeh R, Hadjzadeh MA, Gholamnezhad Z, Aghaei A. The anti-diabetic and antioxidant effects of a combination of Commiphora mukul, Commiphora myrrha and Terminalia chebula in diabetic rats. Avicenna J Phytomed. 2019;9(5):454-464. PubMed
- 39. Orabi SH, Al-Sabbagh ES, Khalifa HK, et al. Commiphora myrrha resin alcoholic extract ameliorates high fat diet induced obesity via regulation of UCP1 and adiponectin proteins expression in rats. Nutrients. 2020;12(3):803. CrossRef PubMed
- 40. Ahmad A, Raish M, Ganaie MA, et al. Hepatoprotective effect of Commiphora myrrha against d-GalN/LPS-induced hepatic injury in a rat model through attenuation of pro inflammatory cytokines and related genes. Pharm Biol. 2015;53(12):1759-1767. CrossRef PubMed
- 41. Anonymous. Standardisation of single drugs of Unani medicine. 1st ed. Part II. New Delhi: CCRUM; 1992. Online
- 42. Usmani MI. Tanqeeh ul Mufradat. 1st ed. New Delhi: Famous Offset Press; 2007:229-230. Online
- 43. Ibn-e-Rushd AWM. Kitab ul Kulliyat. 2nd ed. CCRUM; 1987:79-81.
- 44. Ahmad Al-Undulisi ZA. Al-Jami ul Mufaradat ul Advia Wa Al-Aghziya IB. Vol IV. 2nd ed. New Delhi: CCRUM; 2003:316-320. Online
- 45. Wildner G. Tumors, tumor therapies, autoimmunity and the eye. Autoimmun Rev. 2021;20(9):102892. CrossRef PubMed
- 46. Das S, Pineda G, Goff L, Sobel R, Berlin J, Fisher G. The eye of the beholder: orbital metastases from midgut neuroendocrine tumors, a two institution experience. Cancer Imaging. 2018;18(1):47. CrossRef PubMed
- 47. Domingo RE, Manganip LE, Castro RM. Tumors of the eye and ocular adnexa at the Philippine Eye Research Institute: a 10-year review. Clin Ophthalmol. 2015;9:1239-1247. CrossRef PubMed
- 48. Nahon-Esteve S, Martel A, Maschi C, et al. The molecular pathology of eye tumors: A 2019 update main interests for routine clinical practice. Curr Mol Med. 2019;19(9):632-664. CrossRef PubMed
- 49. Collenette S. The ceropegias of Saudi Arabia. Brit Cact Succ J. 1999;17(4):181-187.
- 50. Chaudhary A, Mossa JS. Contribution to the flora of Saudi Arabia. Pak J Bot. 1991;23(2):257.82. Online
- 51. Gillett J. The genus Trifolium in southern Arabia and in Africa south of the Sahara. Kew Bull. 1952;7(3):367-404. CrossRef
- 52. U.S. Department of Agriculture. Dr. Duke’s phytochemical and ethnobotanical databases. Online. Accessed June 2024.
- 53. Alsanosy R, Alhazmi HA, Sultana S, et al. Phytochemical screening and cytotoxic properties of ethanolic extract of young and mature khat leaves. J Chem. 2020;1-9. CrossRef
- 54. Abdalla AN, Malki WH, Qattan A, Shahid I, Hossain MA, Ahmed M. Chemosensitization of HT29 and HT29-5FU cell lines by a combination of a multi-tyrosine kinase inhibitor and 5FU downregulates ABCC1 and inhibits PIK3CA in light of their importance in Saudi colorectal cancer. Molecules. 2021;26(2):334. CrossRef PubMed
- 55. Abdalla AN, Qattan A, Malki WH, Shahid I, Hossain MA, Ahmed M. Significance of targeting VEGFR-2 and cyclin D1 in luminal-A breast cancer. Molecules. 2020;25(20):4606. CrossRef PubMed
- 56. Chu LI, Berahim Z, Mohamad S, Shahidan WNS, Yhaya MF, Zainuddin SLA. Phytochemical compounds of raw versus methanol-extracted Kelulut, Tualang, and Manuka honeys. Cureus. 2023;15(4):e38297. CrossRef PubMed
- 57. Subramenium GA, Swetha TK, Iyer PM, Balamurugan K, Pandian SK. 5-Hydroxymethyl-2-furaldehyde from marine bacterium Bacillus subtilis inhibits biofilm and virulence of Candida albicans. Microbiol Res. 2018;207:19-32. CrossRef PubMed
- 58. Patil MD, Grogan G, Yun H. Biocatalyzed C-C bond formation for the production of alkaloids. ChemCatChem. 2018;10(21):4783-4804. CrossRef
- 59. Bogaki T, Mitani K, Oura Y, Ozeki K. Effects of ethyl-α-d-glucoside on human dermal fibroblasts. Biosci Biotechnol Biochem. 2017;81(9):1706-1711. CrossRef PubMed
- 60. Aparna V, Dileep KV, Mandal PK, Karthe P, Sadasivan C, Haridas M. Anti-inflammatory property of n-hexadecanoic acid: structural evidence and kinetic assessment. Chem Biol Drug Des. 2012;80(3):434-439. CrossRef PubMed
- 61. Ravi L, Krishnan K. Research article cytotoxic potential of N-hexadecanoic acid extracted from Kigelia pinnata leaves. Asian J Cell Biol. 2017;12(1):20-27. CrossRef
- 62. Fernando IPS, Sanjeewa KKA, Ann Y-S, et al. Apoptotic and antiproliferative effects of Stigmast-5-en-3-ol from Dendronephthya gigantea on human leukemia HL-60 and human breast cancer MCF-7 cells. Toxicol In Vitro. 2018;52:297-305. CrossRef PubMed
- 63. Kakuta S, Usutani S, Sirai M, Nagase M. [Selectivity index]. Jpn J Clin Med. 1997;55(suppl 2):610-613. PubMed
- 64. Pongprayoon U, Soontornsaratune P, Jarikasem S, Sematong T, Wasuwat S, Claeson P. Topical antiinflammatory activity of the major lipophilic constituents of the rhizome of Zingiber cassumunar. Part I: the essential oil. Phytomedicine. 1997;3(4):319-322. CrossRef PubMed
- 65. Cavin A, Hostettmann K, Dyatmyko W, Potterat O. Antioxidant and lipophilic constituents of Tinospora crispa. Planta Med. 1998;64(5):393-396. CrossRef PubMed
- 66. Shin J, Song M-H, Yu J-W, et al. Anticancer potential of lipophilic constituents of eleven shellfish species commonly consumed in Korea. Antioxidants. 2021;10(10):1629. CrossRef PubMed
- 67. Lukevits E, Demicheva L. Biological activity of furan derivatives. Chem Heterocycl Compd. 1993;29(3):243-267. CrossRef
- 68. Shingalapur RV, Hosamani KM, Keri RS. Synthesis and evaluation of in vitro anti-microbial and anti-tubercular activity of 2-styryl benzimidazoles. Eur J Med Chem. 2009;44(10):4244-4248. CrossRef PubMed
- 69. Roleira FM, Varela CL, Costa SC, Tavares-da-Silva EJ. Phenolic derivatives from medicinal herbs and plant extracts: anticancer effects and synthetic approaches to modulate biological activity. Studies Nat Prod Chem. 2018;57:115-156. CrossRef
- 70. Grecco Sdos S, Félix MJ, Lago JH, et al. Anti-trypanosomal phenolic derivatives from Baccharis uncinella. Nat Prod Commun. 2014 Feb;9(2):171-3. PubMed.
- 71. Tracanna MI, Fortuna AM, Cárdenas AV, et al. Anti-leishmanial, anti-inflammatory and antimicrobial activities of phenolic derivatives from Tibouchina paratropica. Phytother Res. 2015;29(3):393-397. CrossRef PubMed
- 72. Woo KW, Kwon OW, Kim SY, et al. Phenolic derivatives from the rhizomes of Dioscorea nipponica and their anti-neuroinflammatory and neuroprotective activities. J Ethnopharmacol. 2014;155(2):1164-1170. CrossRef PubMed