![]() |
Drug Target Insights 2024; 18: 78-83 ISSN 1177-3928 | DOI: 10.33393/dti.2024.3060 ORIGINAL RESEARCH ARTICLE |
![]() |
Levofloxacin induces erythrocyte contraction leading to red cell death
ABSTRACT
Background: Levofloxacin, a fluoroquinolone, is an extensively used antibiotic effective against both positively and negatively staining bacteria. It works by inhibiting bacterial topoisomerase type II and topoisomerase type IV, resulting in impaired DNA synthesis and bacterial cell death. Eryptosis is another term for apoptotic cell death of erythrocyte marked by cell shrinkage, phosphatidylserine (PS) flipping, and membrane blebbing.
Methods: The intent of the present research was to look at the eryptotic effect of levofloxacin by exposing erythrocytes to therapeutical doses (7, 14 µM) of levofloxacin for 48 hours. Cell size evaluation, PS subjection to outside, and calcium channel inhibition were carried out to investigate eryptosis. Oxidative stress generated by levofloxacin was measured as a putative mechanism of eryptosis using glutathione peroxidase (GPx), superoxide dismutase (SOD), and catalase activities. Similarly, hemolysis measurements demonstrated levofloxacin’s cytotoxic effect.
Results: Our findings showed that therapeutic doses of levofloxacin can cause a considerable decline in antioxidant enzymes activities, as well as induce cell shrinkage, PS externalization, and hemolysis in erythrocytes. The role of calcium in triggering erythrocyte shrinkage was also confirmed.
Conclusion: In conclusion, our findings showed that the indicated levofloxacin doses caused oxidative stress, which leads to erythrocyte death via eryptosis and hemolysis. These findings emphasize the importance of using levofloxacin with caution and the need for additional research to mitigate these side effects.
Keywords: Eryptosis, Erythrocyte, Hemolysis, Levofloxacin, Oxidative stress
Received: March 1, 2024
Accepted: July 11, 2024
Published online: October 7, 2024
Corresponding author:
Majeeda Rasheed
email: majeeda.rasheed@kfueit.edu.pk
Drug Target Insights - ISSN 1177-3928 - www.aboutscience.eu/dti
© 2024 The Authors. This article is published by AboutScience and licensed under Creative Commons Attribution-NonCommercial 4.0 International (CC BY-NC 4.0).
Commercial use is not permitted and is subject to Publisher’s permissions. Full information is available at www.aboutscience.eu
Introduction
Levofloxacin is a third-generation antibiotic that belongs to the class of fluoroquinolones. It has more efficacy against positively staining bacteria and uncommon intracellular pathogens than prior generations of fluoroquinolones. Levofloxacin works by inhibiting bacterial type II topoisomerases, particularly the enzymes topoisomerase IV (Topo IV) and bacterial DNA gyrase. In human medicine, levofloxacin is used to treat acute rhinosinusitis, chronic bronchitis, post-exposure inhalational anthrax, hospital-acquired infections and pneumonitis, urinary tract infections, inflammation of the prostate gland, and soft tissue or skin infection. The medicine levofloxacin is on the World Health Organization (WHO)’s list of essential medicines (1,2). In in vivo and in vitro conditions levofloxacin has been demonstrated to have immunomodulatory properties by decreasing the synthesis and secretion of inflammatory cytokines (3). Nausea, vomiting, and diarrhea are among the most common side effects associated with levofloxacin. Central nervous system complications have also been reported from mild dizziness and headache to severe depression, hallucination, and seizures (4). Its widespread usage has been linked to several cases of clinically apparent liver injury. It has been proposed that reactive oxygen species (ROS) produced by the breakdown of vital mitochondrial enzymes, along with those released during RNA processing, gene transcription, and inflammatory processes, causes liver and kidneys to suffer from oxidative stress and cellular damage (5).
The prior work using animal models demonstrated that fluoroquinolones were involved in the formation of reactive oxygen molecules, which is what causes oxidative stress (4). Oxidative stress is a shift in pro-oxidant and antioxidant balance in favor of oxidants, resulting in redox signaling/control disruption and molecular damage (6). ROS can be produced either exogenously by ultraviolet (UV) light, ionizing radiation, pollutants, and nitroaromatics or intracellularly by cytosolic enzyme systems during the course of a variety of physiological and biochemical processes (7). Moreover, levofloxacin was shown to increase lipid peroxidation, NO production, and superoxide dismutase (SOD) activity in cortex, corpus striatum, and hippocampus (8).
The normal lifespan of circulating erythrocytes is 100–120 days owing to erythrocyte aging. Prior to that, erythrocytes are rapidly removed from circulation (9). Eryptosis is the self-destruction of erythrocytes that eliminates old or superfluous erythrocytes from circulation, characterized by erythrocyte membrane perturbation, which results in the dismantling of plasma membrane symmetry and the exposure of phosphatidylserine flipping from the interior to the plasma membrane surface (10,11). Oxidative stress activates several factors that cause erythrocyte suicide, including opening of Ca+2 channels, caspase activation, and the formation of phospholipase A2 (PLA-2)-induced platelet-activating factor (PAF), which stimulate cellular ceramide generation through stimulating sphingomyelinase. Increased cytosolic Ca++ ions and ceramide levels cause phosphatidylserine exposure via membrane scrambling. Phosphatidylserine (PS)-exposed erythrocytes apparently attached to thrombospondin (TSP) are recognized by PS receptors such as PSR-1, and are removed from circulating blood. Furthermore, calcium stimulates Ca-sensitive K+ channel, which results in KCl loss with water that leads to cell shrinkage, and activates calpain, a protease, resulting in cytoskeleton destruction (9,12). A number of xenobiotics have been reported to induce eryptosis associated with the conditions such as anemia, diabetes, cancer, cardiovascular and liver complications, uremia, and chronic kidney disease (13,14). Levofloxacin-induced oxidative stress and anemia are rarely reported in the literature. This research was designed with the intention to look into the involvement of levofloxacin in the induction of eryptosis by oxidative stress. Isolated human red cells were given with therapeutic dosages of levofloxacin in order to evaluate its eryptotic, oxidizing, and hemolyzing or cytotoxic effects on red cells.
Materials and methods
Noninfectious screened blood samples in heparin tubes were generously supplied by the blood bank at the Allied Hospital, Faisalabad. Leukocyte-free erythrocytes were isolated by depleting the white cells and plasma from the whole blood samples following the protocol of Fink et al. The whole blood samples were spun up at 1500 rpm for 15-20 minutes at 21°C and the leukocyte and platelet-rich afloat supernatant was discarded (15). The sorted red blood cells were put into microcentrifuge tubes, and they were incubated for 48 hours at 37°Celsius and pH 7.4 with a 0.4% hematocrit volume in Ringer solution that included sodium chloride 125 mM, potassium chloride 5 mM, magnesium silicate 1 mM, HEPES sodium salt 32 mM, glucose 5 mM, and calcium chloride 1 mM (16). Erythrocytes were exposed to levofloxacin (Getz Pharma Pakistan) at the physiological concentrations (7, 14 µM). Levofloxacin was added from stock solution of 14 mM prepared by dissolving levofloxacin salt in dimethylsulfoxide (DMSO) and later diluted to get the required concentrations for the experiments.
Oxidative stress measurement
Antioxidant enzyme assays (for glutathione peroxidase [GPx], catalase, and SOD) were conducted to assess the stress levels of erythrocytes exposed to levofloxacin.
Superoxide dismutase
SOD activity was determined by using Naveed et al’s protocol (17). The reaction mixture contained nitroblue tetrazolium (0.015 g in 17.5 mL d-H2O), L-methionine (0.222 g in 15 mL d-H2O), Triton-X-100 (0.0375 mL in 17.5 mL H2O), vitamin B2 rib (0.0132 g in 17.5 mL d-H2O), and phosphate buffer of pH 7.4, 0.2 M. The spectrophotometer value of chromophore was taken at 525 nm.
Glutathione peroxidase
Using the methodology described by Sattar et al (18), GPx enzyme activity was evaluated every 20 seconds at 470 nm using spectrophotometer in the reaction mixture containing guaiacol (Sigma-Aldrich; 20 mM), phosphate buffer of pH 5 (50 mM), hydrogen peroxide (40 mM), and 200 µL enzyme extract.
Catalase
The assessment of erythrocyte catalase activity followed the methodology outlined by Mukhtar et al (19). The reaction cocktail composed of phosphate buffer of pH 7.4 (50 mM) and 54 µL of 5.9 nM hydrogen peroxide in 10 mL water. Equal proportions of sample and reaction solution were placed into a multiwell plate (96 wells), and the optical density measured at 240 nm using BioTeK Quant.
Cell size measurements
Variations in cell dimensions were ascertained by calculating the mean cellular volume (MCV) for both the controlled and levofloxacin-treated erythrocytes. The MCV was calculated using an HumaCount 5D hematology analyzer (Human GmbH, Wiesbaden, Germany), which provided estimated information on red blood cell volume and shape and size (20).
Phosphatidylserine content
Concentration of externalized PS in levofloxacin-treated erythrocytes was measured using a human PS enzyme-linked immunosorbent assay (ELISA) kit (Elabscience, Beijing, People’s Republic of China) according to the manufacturer’s instructions. Absorbance was read at wavelength of 450 nm on the BioTeK μ Quant microplate reader and expressed as PS equivalents (mg/mL) (21).
Confirmation of the role of Ca++ ions
To validate the functional role of Ca++ in the initiation of eryptosis, the MCV of erythrocytes was evaluated using a hematology analyzer. Erythrocytes were treated with levofloxacin doses and 10 µM of a calcium entry blocker (amlodipine) to ensure the involvement of calcium ions in levofloxacin-induced eryptosis (22).
Measurement of hemolysis percentage
To validate levofloxacin’s hemolytic effect on erythrocytes, controlled and levofloxacin-treated red cell samples were centrifuged at 400g for 3 minutes at 25°C to get the supernatant. The concentration of hemoglobin in supernatants was measured at 405 nm using LMSP-V310 visible spectrophotometer; 100% hemolysis was defined as the optical density of erythrocyte supernatant lysed in distilled water (23).
Statistical analysis
The findings were presented with a mean standard error of mean (± SEM). Analysis of variance (ANOVA) with Tukey’s test as a follow-up test was used for the statistical study where necessary (24). Statistical significance was considered as p-value <0.05.
Results
The purpose of this study was to analyze levofloxacin’s eryptotic effects by implementing the oxidative stress induction that is one of the eryptosis-stimulating mechanisms. To validate this, the antioxidant enzymatic activities, erythrocyte size, exposing of PS at the cell exterior, hemolytic activity, and substantiating the involvement of calcium in triggering the programmed cell death of erythrocytes were all evaluated. The findings of the research are showcased in graphs, featuring mean values ±SEM with clear indications of statistical significance. The antioxidant enzyme activities in levofloxacin-treated and -nontreated cells (control group) were measured to see if levofloxacin induces oxidative stress. Figure 1 shows a notable decrease in SOD activity at 7 and 14 μM levofloxacin compared to control cells. Figure 2 shows that 48 hours treatment with the mentioned drug concentrations resulted in a statistically substantial drop in GPx activity at 7 and 14 μM doses compared with control groups. Similarly, Figure 3 shows that 48 hours of treatment with levofloxacin results in a relatively moderate decline at 7 µM and highly significant decline at 14 µM in catalase activity.
A substantial and statistically significant decrease in the MCV of red blood cells was observed after treatment with 7 and 14 µM concentrations of levofloxacin for 48 hours (Fig. 4). Increased mean cell volume could be attributed to membrane bleb formation caused by levofloxacin-induced oxidative damage.
Results of PS exposure assay showed least absorbance in the control group, which indicates minor PS externalization, while levofloxacin-exposed treatment groups showed increasing absorbance (p < 0.001) in both 7 and 14 µM concentrations, reflecting increased PS exposure and suggesting higher levels of eryptosis. The standard curve was generated using the absorbance values of the known PS standards, which showed a linear relationship between absorbance and PS concentration (Fig. 5).
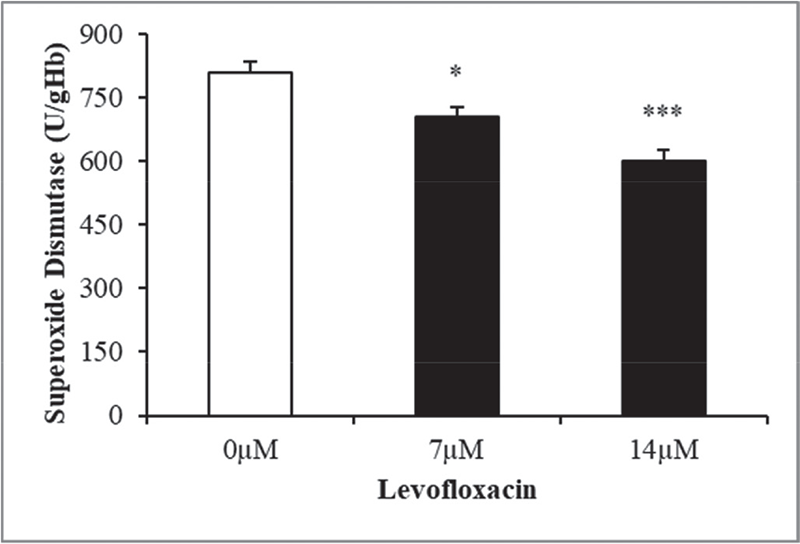
FIGURE 1 - The effect of levofloxacin on superoxide dismutase activity in erythrocytes exposed to levofloxacin (measured in U/g Hb). Variations in erythrocyte responses (n = 15) are visible after 48 hours of incubation in Ringers’ solution with varying concentrations of levofloxacin in black bars, with asterisks *(p < 0.05) and ***(p < 0.0001) on the error bars indicating significant differences from the absence of levofloxacin in white bar (ANOVA). ANOVA = analysis of variance.
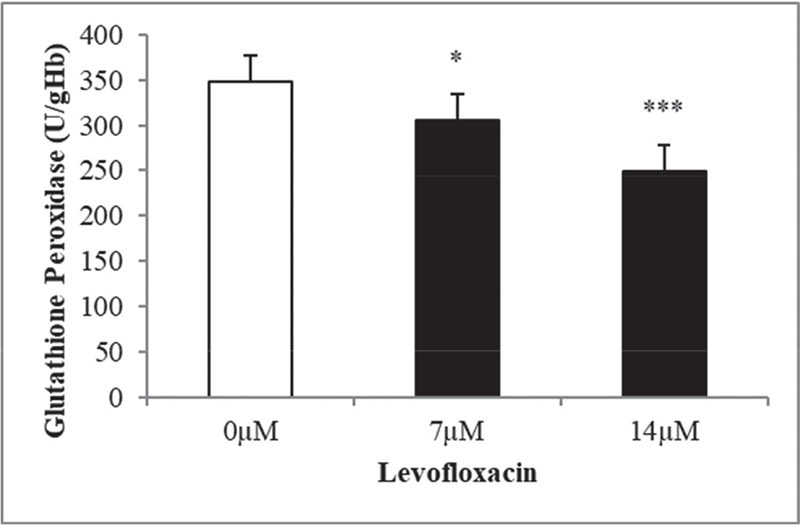
FIGURE 2 - The effect of levofloxacin on glutathione peroxidase activity in erythrocytes exposed to levofloxacin (measured in U/g Hb). Variations in erythrocyte responses (n = 15) are visible after 48 hours of incubation in Ringer’s solution with varying concentrations of levofloxacin in black bars, with asterisks *(p < 0.05) and ***(p < 0.0001) on the error bars indicating significant distinctness from the absence of levofloxacin in white bar (ANOVA). ANOVA = analysis of variance.
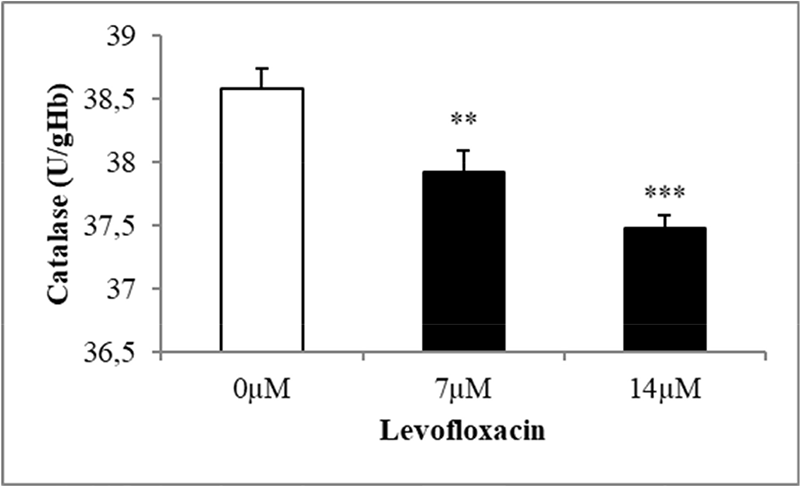
FIGURE 3 - The effect of levofloxacin on catalase activity in erythrocytes exposed to levofloxacin (measured in U/g Hb). Variations in erythrocyte responses (n = 15) are visible after 48 hours of incubation in Ringer’s solution with varying concentrations of levofloxacin in black bars, with asterisks **(p < 0.01) and ***(p < 0.0001) on the error bars indicating significant differences from the absence of levofloxacin in white bar (ANOVA). ANOVA = analysis of variance.
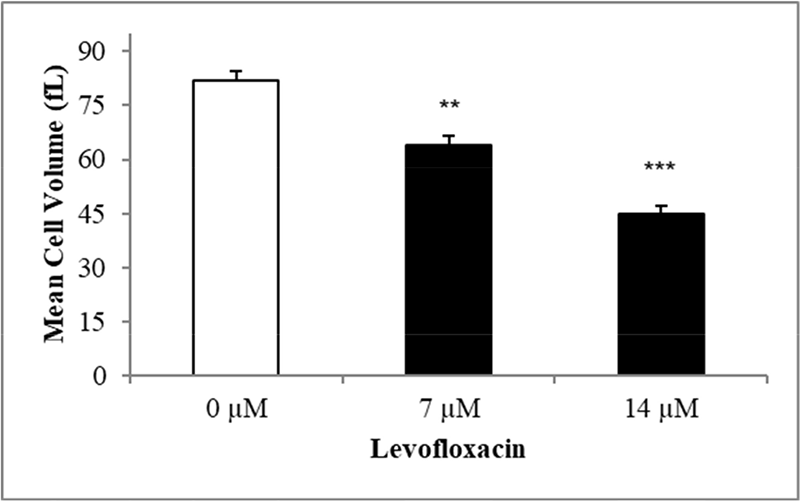
FIGURE 4 - The effect of levofloxacin on erythrocyte mean cellular volume (fL). Mean ± SEM (n = 10) of erythrocytes incubated in Ringer’s solution for 48 hours without levofloxacin in white bar and with levofloxacin concentrations (7, 14 µM) in black bars. The Y-axis represents ±SEM. **(p < 0.01) and ***(p < 0.001) indicate a statistically significant distinction in groups treated with levofloxacin in comparison to the absence of levofloxacin (ANOVA). ANOVA = analysis of variance; SEM = standard error of the mean.
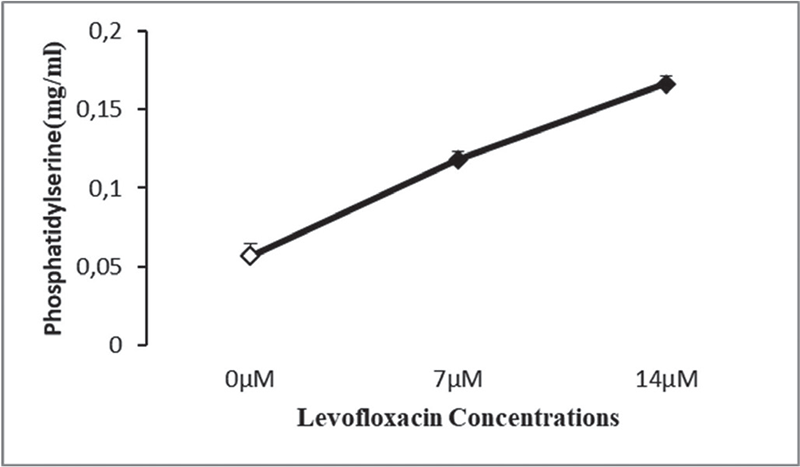
FIGURE 5 - Effect of levofloxacin on phosphatidylserine content following incubation in Ringer’s solution for 48 hours in the absence (control group) and presence of levofloxacin (7, 14 µM).
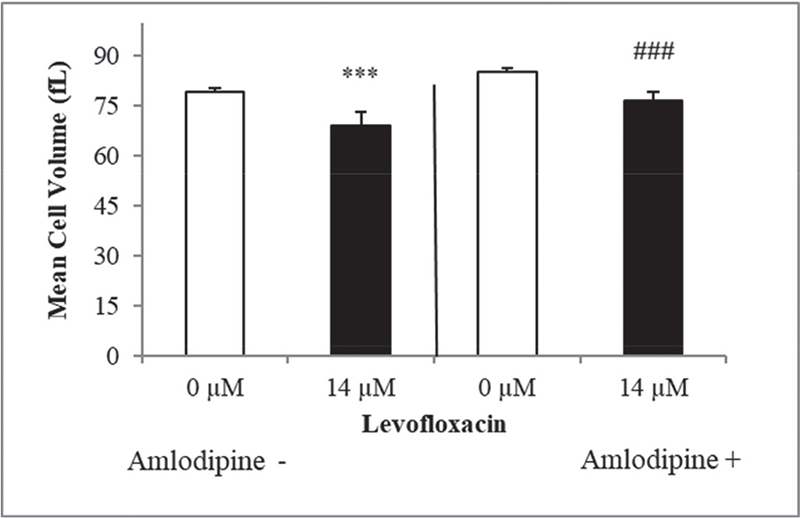
FIGURE 6 - Measurement of erythrocyte mean cellular volume in the presence and absence of amlodipine following exposure to levofloxacin, represented by arithmetic mean ± SEM (n = 10) for erythrocyte incubated in Ringer’s solution, with 14 μM levofloxacin (in black bar) or without (in white bars) demonstrating a decrease in cell dimensions subsequent to amlodipine 10 μM treatment (ANOVA) as indicated by ***(p < 0.001), ###(p < 0.001). ANOVA = analysis of variance; SEM = standard error of the mean.
The calcium channel blocker amlodipine was employed to verify the effect of Ca++ in the elicitation of oxidative stress initiated by eryptosis in triggering the formation of membrane blebs. Figure 6 showed noticeable rise in erythrocyte cell size dimensions when compared to cells that were free of amlodipine, which is most likely due to calcium entrance inhibition in the cells. This experiment revealed no shrinkage in the size of erythrocytes, due to the blockade of Ca++ channels confirming the significance of calcium in causing erythrocyte cell death. Figure 7 shows the findings of % hemolysis in levofloxacin-treated cells to confirm the hemolytic activity of levofloxacin. Figure 6 shows an increase in % hemolysis after 2 days of levofloxacin (14 µM) exposure of erythrocytes.
In accordance with our research it was concluded that therapeutic dosages (7, 14 µM) of levofloxacin may elevate the rate of erythrocyte elimination from the blood stream due to oxidative stress and calcium influx, leading to eryptosis and hemolyzing the red cells.
Discussion
The intent of this study was to find the impact caused by levofloxacin on oxidative stress status and cell death of red blood cells. This objective of the study was achieved by investigating the antioxidant enzymes’ status, cell dimensions, and the role of calcium in causing the programmed cell death of red cells. The levofloxacin concentrations (7, 14 μM) employed in this investigation were the plasma concentration reported by Fish and Chow (25). One of the primary drivers of eryptosis is oxidative stress (14), and increasing oxidative damage leads to a drop in the activities of free radical scavenger enzymes (26). The impairment in enzymatic activity of SOD could be predictive of the heightened superoxide radical concentrations, as SOD efficiently catalyzes the conversion of oxygen into H2O2. A decrease in SOD activity was also evaluated by repeated oral administration of levofloxacin and other fluoroquinolones in rabbits (27). Similar effect was also reported on hepatic and renal tissues of rat due to exposure of levofloxacin (28).
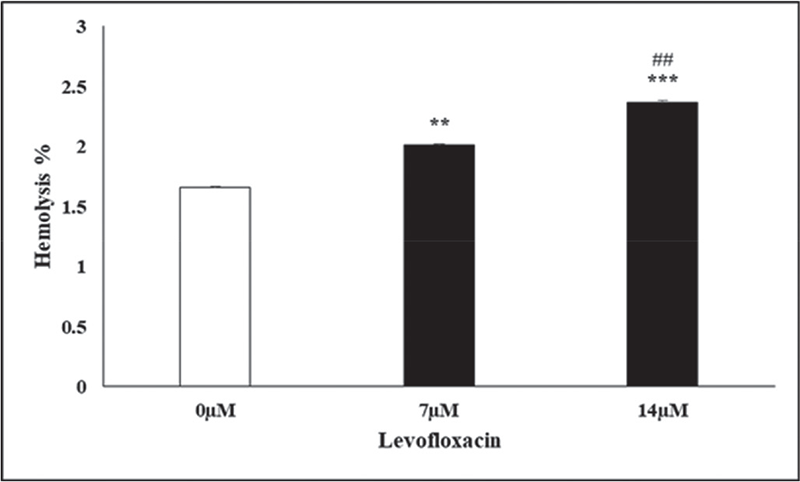
FIGURE 7 - The % hemolysis effect of levofloxacin in red cells is shown. The arithmetic mean ± SEM (n = 12) for erythrocytes incubated in Ringer’s solution with 7 and 14 µM levofloxacin (in black bars) and without levofloxacin (in white bar) for 48 hours is presented, with the Y-axis indicating SEM. **(p < 0.01) and ***(p < 0.001) served to emphasize notable differences in comparison to the absence of levofloxacin at 7 and 14 µM, respectively (ANOVA), while ## reflects distinctions between the % hemolysis at 7 and 14 µM levofloxacin concentration. ANOVA = analysis of variance; SEM = standard error of the mean.
The experiment revealed a moderate (at 7 µM) and notable reduction (at 14 µM) in GPx activity, which might be blamed on the formation of ROS. In a related research under stress red cells, a substantial endogenous rate of H2O2 synthesis from hemoglobin autoxidation was observed. GPx catalyzed a reaction by which glutathione is oxidized to detoxify hydrogen peroxide. Increasing oxidative stress ultimately leads to demise in GPx activity. The reduced enzyme activity is due to a decrease in glutathione concentration (29). Calderón-Salinas et al (30) reported that oxidative stress made the red cell to expose PS outside, which also decreases erythrocyte count.
Catalase activity was also seen to be declined in the present study in levofloxacin-treated erythrocytes compared with controlled groups. The decreased catalase activity might be due to peroxidative damage to lipids and injury to cellular components by levofloxacin-induced oxidative insult. Parallel results were also reported by Khan et al (27) and Farid and Hegazy (31).
Erythrocyte shrinkage and PS externalization on the erythrocyte membrane are two eryptotic markers. The high PS exposure on the outer leaflet of erythrocyte’s plasma membrane in levofloxacin-treated erythrocytes indicates that levofloxacin exposure leads to increased eryptosis. Obtained data showed significant increase in PS externalization in levofloxacin-treated erythrocytes while control group showed negligible PS exposure. Thus, increasing concentrations of levofloxacin lead to higher PS exposure, which is the main indicator of eryptosis (16). A decrease in mean cell volume confirms stimulated eryptosis (24). The activation of a calcium influx channel in red blood cells by oxidative stress results in considerable erythrocyte shrinkage (13). This action is believed to be triggered by Ca2+-dependent K+ channel activation in the erythrocyte’s membrane, which results in increased polarization of membrane and eventual K+ loss followed by Cl− in the erythrocyte (32). Our results showed apparent difference in cell shrinkage in amlodipine-treated and -nontreated erythrocytes, which confirms the role of calcium ions in triggering characteristic cell shrinking of eryptotic erythrocytes.
Eryptosis has an important physiological influence on the disposal of faulty erythrocytes prior to hemolysis (33). Hemoglobin flows out from hemolyzed cells that may be contrarily filtered in the glomerulus and clog the renal tubules (34). Our results showed a high increase in % hemolysis (Fig. 6) after 48 hours of levofloxacin exposure to erythrocytes at 7 and 14 µM. The release of erythrocyte contents, particularly hemoglobin, during hemolysis reduces NO bioavailability. This may lead to vasomotor instabilities, systemic vasoconstriction, and endothelial dysfunction, as well as crucial clinical problems such as hypertension, respiratory issues, cardiovascular disorders, impaired renal function, inflammation, coagulation, and vulnerability to infections (35).
Data Availability Statement
The data that support the findings of this study are available from the corresponding author upon reasonable request.
Acknowledgments
Acknowledge the support and guidance of the researchers and mentors at the Department of Biochemistry, University of Agriculture Faisalabad.
Disclosures
Financial support: The author(s) received no financial support for the research, authorship, and/or publication of this article.
Conflict of interest: The authors declare no conflict of interest.
References
- 1. Trespalacios-Rangél AA, Otero W, Arévalo-Galvis A, Poutou-Piñales RA, Rimbara E, Graham DY. Surveillance of levofloxacin resistance in Helicobacter pylori isolates in Bogotá-Colombia (2009-2014). PLoS One. 2016;11(7):e0160007. CrossRef PubMed
- 2. Sitovs A, Sartini I, Giorgi M. Levofloxacin in veterinary medicine: a literature review. Res Vet Sci. 2021;137:111-126. CrossRef PubMed
- 3. Zusso M, Lunardi V, Franceschini D, et al. Ciprofloxacin and levofloxacin attenuate microglia inflammatory response via TLR4/NF-kB pathway. J Neuroinflammation. 2019;16(1):148. CrossRef PubMed
- 4. Talla V, Veerareddy P. Oxidative stress induced by fluoroquinolones on treatment for complicated urinary tract infections in Indian patients. J Young Pharm. 2011;3(4):304-309. CrossRef PubMed
- 5. Abdullah RA, Taee FD, Thanoon IA. Effect of levofloxacin on some body tissues in mice. Iraqi J Vet Sci. 2021;35(1):109-111. CrossRef
- 6. Sies H. The concept of oxidative stress after 30 years. In: Gelpi R, Boveris A, Poderoso J, eds. Biochemistry of oxidative stress. Advances in biochemistry in health and disease. vol 16. Cham: Springer; 2016. CrossRef
- 7. Haider K, Haider MR, Neha K, Yar MS. Free radical scavengers: an overview on heterocyclic advances and medicinal prospects. Eur J Med Chem. 2020;204:112607. PubMed PMID:32721784 CrossRef PubMed
- 8. Mani S, Tyagi S, Pal KV, et al. Drug-induced oxidative stress and cellular toxicity. In: Kesari KK, Jha NK, eds. Free radical biology and environmental toxicity. Springer; 2022: 73-113. CrossRef
- 9. Lang F, Jilani K, Lang E. Therapeutic potential of manipulating suicidal erythrocyte death. Expert Opin Ther Targets. 2015;19(9):1219-1227. CrossRef PubMed
- 10. Pyrshev KA, Klymchenko AS, Csúcs G, Demchenko AP. Apoptosis and eryptosis: striking differences on biomembrane level. Biochim Biophys Acta Biomembr. 2018;1860(6):1362-1371. CrossRef PubMed
- 11. Dreischer P, Duszenko M, Stein J, Wieder T. Eryptosis: programmed death of nucleus-free, iron-filled blood cells. Cells. 2022 Feb 1;11(3):503. CrossRef PubMed
- 12. Lang F, Lang KS, Lang PA, Huber SM, Wieder T. Mechanisms and significance of eryptosis. Antioxid Redox Signal. 2006;8(7-8):1183-1192. CrossRef PubMed
- 13. Lang F, Gulbins E, Lerche H, Huber SM, Kempe DS, Foller M. Eryptosis, a window to systemic disease. Cell Physiol Biochem. 2008;22(5-6):373-380. CrossRef PubMed
- 14. Pretorius E, du Plooy JN, Bester J. A comprehensive review on eryptosis. Cell Physiol Biochem. 2016;39(5):1977-2000. CrossRef PubMed
- 15. Fink M, Al Mamun Bhuyan A, Zacharopoulou N, Lang F. Stimulation of eryptosis, the suicidal erythrocyte death, by costunolide. Cell Physiol Biochem. 2018;50(6):2283-2295. CrossRef PubMed
- 16. Bissinger R, Malik A, Jilani K, Lang F. Triggering of erythrocyte cell membrane scrambling by salinomycin. Basic Clin Pharmacol Toxicol. 2014;115(5):396-402. CrossRef PubMed
- 17. Naveed A, Jilani K, Siddique AB, et al. Induction of erythrocyte shrinkage by omeprazole. Dose Response. 2020 Aug 3;18(3):1559325820946941. CrossRef PubMed
- 18. Sattar T, Jilani K, Parveen K, Mushataq Z, Nawaz H, Khan MAB. Induction of erythrocyte membrane blebbing by methotrexate-induced oxidative stress. Dose Response. 2022 Apr 13;20(2):15593258221093853. CrossRef PubMed
- 19. Mukhtar F, Jilani K, Bibi I, Mushataq Z, Bari Khan MA, Fatima M. Stimulation of erythrocyte membrane blebbing by bifenthrin induced oxidative stress. Dose Response. 2022 Mar 3;20(1):15593258221076710. CrossRef PubMed
- 20. Ilyas S, Jilani K, Sikandar M, et al. Stimulation of erythrocyte membrane blebbing by naproxen sodium. Dose Response. 2020;18(1):1559325819899259. CrossRef PubMed
- 21. Burger D, Turner M, Xiao F, et al. High glucose increases the formation and pro-oxidative activity of endothelial microparticles. Diabetologia. 2017;60:1791-1800. CrossRef
- 22. Shabir K, Jilani K, Zbidah M, et al. Triggering of erythrocyte membrane blebbing by ciprofloxacin. Acta Pol Pharm. 2019;76(5):901-906. CrossRef
- 23. Mischitelli M, Jemaà M, Almasry M, Faggio C, Lang F. Triggering of erythrocyte cell membrane scrambling by emodin. Cell Physiol Biochem. 2016;40(1-2):91-103. CrossRef PubMed
- 24. Lupescu A, Bissinger R, Jilani K, Lang F. Triggering of suicidal erythrocyte death by celecoxib. Toxins. 2013;5(9):1543-1554. CrossRef
- 25. Fish DN, Chow AT. The clinical pharmacokinetics of levofloxacin. Clin Pharmacokinet. 1997;32(2):101-119. CrossRef PubMed
- 26. Vaziri ND, Dicus M, Ho ND, Boroujerdi-Rad L, Sindhu RK. Oxidative stress and dysregulation of superoxide dismutase and NADPH oxidase in renal insufficiency. Kidney Int. 2003;63(1):179-185. CrossRef PubMed
- 27. Khan AM, Rampal S, Sood NK. Effect of repeated oral administration of levofloxacin, enrofloxacin, and meloxicam on antioxidant parameters and lipid peroxidation in rabbits. Hum Exp Toxicol. 2017;36(1):42-50. CrossRef PubMed
- 28. Olayinka ET, Ore A, Ola OS. Influence of different doses of levofloxacin on antioxidant defense systems and markers of renal and hepatic dysfunctions in rats. Adv Toxicol. 2015. CrossRef
- 29. Waggiallah H, Alzohairy M. The effect of oxidative stress on human red cells glutathione peroxidase, glutathione reductase level, and prevalence of anemia among diabetics. N Am J Med Sci. 2011;3(7):344-347. CrossRef
- 30. Calderón-Salinas JV, Muñoz-Reyes EG, Guerrero-Romero JF, et al. Eryptosis and oxidative damage in type 2 diabetic mellitus patients with chronic kidney disease. Mol Cell Biochem. 2011;357(1-2):171-179. CrossRef PubMed
- 31. Farid AS, Hegazy AM. Ameliorative effects of Moringa oleifera leaf extract on levofloxacin-induced hepatic toxicity in rats. Drug Chem Toxicol. 2020;43(6):616-622. CrossRef PubMed
- 32. Lang KS, Duranton C, Poehlmann H, et al. Cation channels trigger apoptotic death of erythrocytes. Cell Death Differ. 2003;10(2):249-256. CrossRef PubMed
- 33. Charras GT, Coughlin M, Mitchison TJ, Mahadevan L. Life and times of a cellular bleb. Biophys J. 2008 Mar 1;94(5):1836-1853. CrossRef PubMed
- 34. Harrison HE, Bunting H, Ordway NK, Albrink WS. The pathogenesis of the renal injury produced in the dog by hemoglobin or methemoglobin. J Exp Med. 1947;86(4):339-356. CrossRef PubMed
- 35. Rapido F. The potential adverse effects of haemolysis. Blood Transfus. 2017 May;15(3):218-221. CrossRef PubMed