![]() |
Drug Target Insights 2024; 18: 8-19 ISSN 1177-3928 | DOI: 10.33393/dti.2024.3019 REVIEW |
![]() |
The dark side of drug repurposing. From clinical trial challenges to antimicrobial resistance: analysis based on three major fields
ABSTRACT
Drug repurposing is a strategic endeavor that entails the identification of novel therapeutic applications for pharmaceuticals that are already available in the market. Despite the advantageous nature of implementing this particular strategy owing to its cost-effectiveness and efficiency in reducing the time required for the drug discovery process, it is essential to bear in mind that there are various factors that must be meticulously considered and taken into account. Up to this point, there has been a noticeable absence of comprehensive analyses that shed light on the limitations of repurposing drugs. The primary aim of this review is to conduct a thorough illustration of the various challenges that arise when contemplating drug repurposing from a clinical perspective in three major fields—cardiovascular, cancer, and diabetes—and to further underscore the potential risks associated with the emergence of antimicrobial resistance (AMR) when employing repurposed antibiotics for the treatment of noninfectious and infectious diseases. The process of developing repurposed medications necessitates the application of creativity and innovation in designing the development program, as the body of evidence may differ for each specific case. In order to effectively repurpose drugs, it is crucial to consider the clinical implications and potential drawbacks that may arise during this process. By comprehensively analyzing these challenges, we can attain a deeper comprehension of the intricacies involved in drug repurposing, which will ultimately lead to the development of more efficacious and safe therapeutic approaches.
Keywords: Thalidomide, Levofloxacin, Minocycline, Doxycycline, Azithromycin, Hydroxychloroquine
Received: January 2, 2024
Accepted: April 18, 2024
Published online: May 10, 2024
Corresponding author:
Majd M. Alsaleh
email: majd.alsaleh@bau.edu.jo
Drug Target Insights - ISSN 1177-3928 - www.aboutscience.eu/dti
© 2024 The Authors. This article is published by AboutScience and licensed under Creative Commons Attribution-NonCommercial 4.0 International (CC BY-NC 4.0).
Commercial use is not permitted and is subject to Publisher’s permissions. Full information is available at www.aboutscience.eu
Introduction
Drug repurposing, otherwise referred to as drug repositioning, is a tactical attempt encompassing the identification of novel therapeutic applications for already existing pharmaceuticals. It presents itself as a highly advantageous strategy due to its cost-effective nature and ability to save time in the drug discovery process, all while mitigating the risks of failure as opposed to customary approaches (1). Drug repurposing is an extremely advantageous technique in the field of pharmaceutical research because it capitalizes on the unintentional off-target effects of extant medications (2). This novel approach includes the use of pharmaceuticals that have previously been approved, drugs that have been declared ineffective in clinical trials, and drugs that have been removed from the market for a variety of reasons (3). By venturing into the realm of drug repurposing, researchers can unlock a vast reservoir of therapeutic potential that has hitherto remained untapped.
However, the question posed by several readers, researchers, and patients is as follows: “Have any drugs that have been repurposed actually received approval for their new indications?” At first, one may find oneself engaged in confident mental processes, but with an impassive countenance, while seeking to recall enough examples to confirm the current reaction. Still, a memorable case, such as thalidomide, will spring to mind (4). The drug, infamously recognized in its devastating original form as a sedative and curative for morning sickness, returned to the medical world for the treatment of leprosy and, subsequently, multiple myeloma (5). It is worth noting that the discovery of thalidomide’s efficacy in treating leprosy, specifically erythema nodosum leprosum, was a serendipitous occurrence (6). The story behind this accidental discovery is recounted in the book Dark Remedy. In this tale, a physician administered the only sedative available in the hospital’s pharmacy, which had not been previously attempted, to a suffering leprosy patient. Astonishingly, the drug had a dramatic and unexpected effect on the patient’s condition (7). This is a perfect example of repurposing a medicine that was authorized and then abandoned (and the method in which it was abandoned) to treat a completely other ailment (8). Another example of drug repurposing that is widely recognized is sildenafil, which is also commonly known as Viagra. Initially, this medication was prescribed for the treatment of arterial narrowness, hypertension, and heart disease in both humans and animals. However, it was fortuitously discovered that sildenafil has beneficial effects on erectile tissue dysfunction in male genital organs (9). Following its approval by the US Food and Drug Administration (FDA) in 1998, sildenafil has brought about a significant transformation in the management of erectile dysfunction (ED) and has contributed to a deeper comprehension of the underlying scientific principles behind ED and its impact on men’s overall well-being (10). In addition to its application in treating ED, sildenafil has also demonstrated efficacy in addressing pulmonary arterial hypertension (PAH) (11). Early investigations and clinical trials have explored the potential favorable impacts of sildenafil on various organs, including the heart, liver, kidney, brain, and intestines (12). Nevertheless, further research is necessary in order to fully comprehend the effects of sildenafil on different diseases and organs (13). Despite the numerous obstacles and unsuccessful attempts that we have elaborated on in the preceding sections, there have been instances of drug repurposing that have achieved success. Notable examples include the utilization of zidovudine (azidothymidine, AZT) as a therapeutic agent against human immunodeficiency virus (HIV) (14) and the repurposing of tocilizumab for the treatment of COVID-19 (15). The practice of repurposing drugs has demonstrated its effectiveness in the development of therapeutic strategies for various diseases and holds promise in addressing rare and difficult conditions (3). This approach entails a combination of experimental and computational methods, leveraging existing safety data and redirecting the application of drugs based on validated target molecules (16). The utilization of Application Programming Interfaces (APIs) in drug repurposing is increasingly being acknowledged as a promising technique for expediting the process of drug discovery and development (17). Integrative approaches that extract data from multiple sources through APIs offer the advantages of adaptability, reusability, and transparency (18). One particular study outlined a strategy for ligand-based in silico drug repurposing utilizing the analytical platform KNIME, which entailed targeted data retrieval, data curation, and substructure searches in DrugBank and other databases (19). Another study developed a fully automated and parameter-free virtual screening server called DrugRep, which conducted molecular structure construction, docking, similarity comparison, and binding affinity screening in a completely autonomous manner (20). These computational tools furnish researchers with user-friendly interfaces and interactive predictions, thereby enhancing the accessibility and efficiency of drug repurposing (21). Through the optimization of the therapeutic potential of already existing drugs, drug repurposing enhances the likelihood of attaining successful outcomes and offers a means to promptly identify effective treatments (22). Thinking deeply, we can conclude that the development of repurposed medications necessitates the application of creativity and innovation in designing the development program, as the body of evidence differs for each individual case not depending on the chance (23).
Several research studies have focused their attention on investigating and understanding the potential advantages and positive outcomes associated with the practice of drug repurposing. However, it is worth noting that, up until now, there has been a lack of comprehensive reviews that shed light on the potential drawbacks and negative aspects of drug repurposing. Therefore, the main aim of this particular review is to provide an in-depth analysis and exploration of the various challenges that arise when considering drug repurposing from a clinical perspective in the fields of cardiovascular disease, cancer, and diabetes. Additionally, the primary objective of this analysis is to underscore the heightened risk associated with the development of AMR that arises from the utilization of antibiotics for purposes other than their original intended use, whether it be for treating various infectious diseases or noninfectious conditions that deviate from their initial approved indications.
Drug repurposing challenges in clinical view
The process of drug repurposing has a number of drawbacks. One key barrier is the pharmaceutical industry’s restricted focus on diseases that provide more financial rewards, lowering the amount of repurposing chances for orphan diseases and neglected tropical disorders (24). Furthermore, there are legal concerns surrounding repurposed medications, including restricted patent coverage and obstacles encountered during the execution of clinical trials, both of which decrease the likelihood of success (25). It is imperative to address additional challenges that require attention, such as the generation of false-positive signals during data mining and the vulnerability of hypothesis validation to bias and confounding (26). Moreover, the absence of clear regulatory guidance poses yet another hindrance in the field of drug repurposing (27). Despite the inherent advantages of this technique, such as cheaper costs and faster time frames, it is crucial to recognize substantial failures found during clinical trials, when the repurposed medicine may fail to exhibit a good balance of benefits and risks (28). Consequently, a comprehensive analysis of these challenges and limitations must precede the pursuit of drug repurposing strategies.
Challenges in cardiovascular endeavor
The clinical perspective on drug repurposing has faced numerous challenges and trials that have not been successful. Despite the potential benefits of repurposing current drugs for unique applications, there are specific limitations that hinder success in this endeavor. These limitations encompass a lack of resources, difficulties with accessing data, and concerns regarding personnel, all of which can impede the process of drug profiling (29). Within the realm of cardiovascular disease, repurposing anti-inflammatory medicines has proven to be challenging due to unforeseen consequences and the necessity for prolonged therapy (30). In a study conducted by Ridker et al (31), it was found that the repurposing of the therapeutic agent canakinumab possesses the capacity to induce unfavorable outcomes, which encompasses the occurrence of neutropenia as well as an elevated susceptibility to mortality specifically attributed to infection or sepsis, as illustrated in Figure 1.
Another instance exemplified in Figure 1 arises when etanercept is employed as a repurposed therapeutic agent for the management of cardiovascular ailments. The clinical trials were terminated prematurely due to the conspicuous absence of any discernible benefit (32). In the same domain, another agent, colchicine, which had been repurposed, was subjected to different clinical trials as illustrated in Figure 2. These trials brought to light a noteworthy escalation in adverse gastrointestinal symptoms and an increased vulnerability to pneumonia (33,34). Moreover, it is important to note that there was no significant discrepancy observed in major adverse cardiovascular events (MACEs) between the group administered with colchicine and the group administered with a placebo (35). However, it is worth mentioning that the incidence of total mortality exhibited an augmentation in the colchicine group, primarily due to sepsis (35). Additionally, in a study conducted by Mewton et al (36), it was observed that there was no substantial reduction in the size of the infarct at the conclusion of a 5-day period (36). Within the same clinical trial, there was no significant decrease in MACE during the 3-month follow-up period. Furthermore, no disparity was discovered in the levels of inflammatory markers, including white blood cell count and C-reactive protein (CRP), within the initial 48 hours.
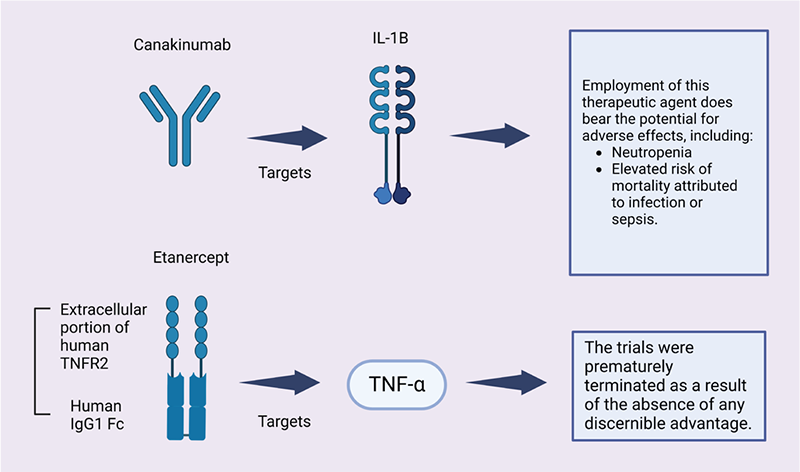
FIGURE 1 - Examples on drug repurposed in cardiovascular endeavor. Canakinumab (ACZ885, Ilaris), a monoclonal antibody targeting human anti-interleukin-1β, has been developed by Novartis (37). In June 2009, the US Food and Drug Administration granted approval for its use in the treatment of familial cold autoinflammatory syndrome and Muckle-wells syndrome, both of which pertain to cryopyrin-associated periodic syndromes, a group of inflammatory diseases. However, the attempt by Novartis to repurpose this drug, which has been approved for rare inflammatory diseases, for a cohort of heart attack survivors has been rejected by the US Food and Drug Administration, as announced by the Swiss pharmaceutical company (38). It is worth noting that although the scientific community highly praised the results obtained from Novartis’ Cantos study, some cardiovascular experts were less enthusiastic. These experts concluded that the benefits of this medication were not significant enough to warrant expanding its approved use to include routine treatment of cardiac patients. A noteworthy example supporting the viewpoint of these cardiovascular experts is the study conducted by Ridker et al (31), which demonstrated that the repurposing of this therapeutic agent yielded various adverse effects, as depicted in this figure.
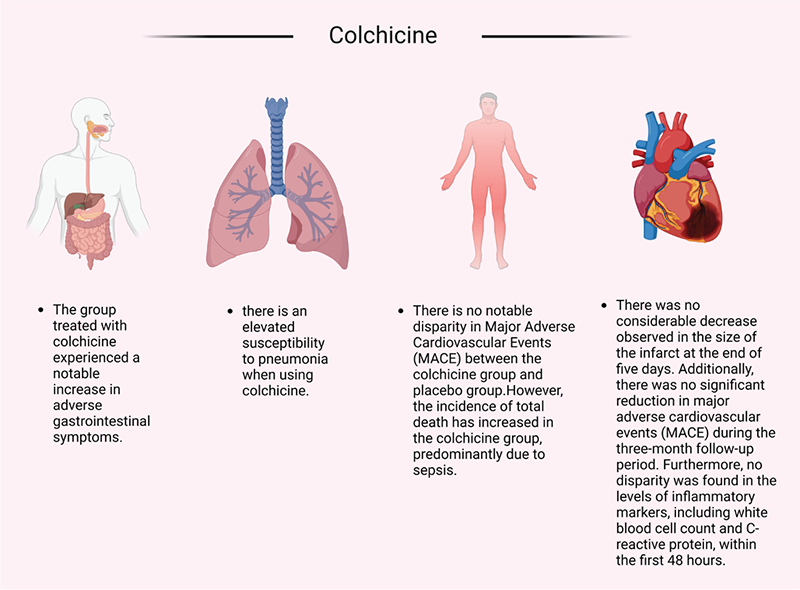
FIGURE 2 - Challenges in clinical trials using colchicine. In the year 2009, the US FDA granted approval for the use of colchicine in the treatment of familial Mediterranean fever (FMF) and the prevention and management of gout attacks (41). Detailed investigations into the mechanisms of action have revealed that colchicine exhibits its effects by binding to microtubules, thereby exerting an influence on numerous cellular processes (42). Additionally, colchicine has been found to impede the expression of various inflammatory cytokines (43). The concept that chronic inflammation contributes to the development of cardiovascular diseases, such as coronary artery disease (CAD), is currently gaining considerable scientific attention (44). The distinctive anti-inflammatory mechanism of colchicine has consequently prompted further investigations in the domain of cardiovascular medicine (45). At present, the utilization of colchicine in cardiovascular therapy remains quite limited (46,47). This constraint may be attributed to the adverse effects observed in clinical trials focused on cardiovascular conditions. These adverse effects, as depicted in the figure, encompass gastrointestinal disorders (33), pneumonia (34), and sepsis, which may ultimately lead to an increased incidence of overall mortality (35). Furthermore, individuals treated with this medication did not exhibit any notable differences in terms of infarct size or levels of inflammatory markers, including white blood cell count and C-reactive protein, within the initial 48-hour period. Moreover, a significant decrease in the occurrence of MACE was not observed during the 3-month follow-up period (36).
On the other hand, etanercept is a pharmaceutical agent employed for the purpose of managing and addressing autoimmune disorders, such as plaque psoriasis, rheumatoid arthritis, psoriatic arthritis, juvenile idiopathic arthritis, and ankylosing spondylitis. It belongs to the class of biologic fusion proteins categorized as tumor necrosis factor (TNF) blockers (39). Although the approval of systemic etanercept for adults occurred in 2004, the FDA declined to approve its use in children with psoriasis in 2018 (40). However, the FDA later revised their risk-benefit assessment in light of a better understanding of the disease burden, the unmet medical needs, and the impact of off-label use in children with psoriasis. Consequently, in 2016, this led to the approval of etanercept as the first systemic biologic product for the treatment of moderate to severe psoriasis in children aged 4-17. Despite these findings, some researchers have attempted to repurpose this drug for the treatment of cardiovascular diseases. An example of such an attempt is seen in a clinical trial, depicted in the accompanying figure, which was terminated due to the absence of any significant advantages (32).
Another drug repurposed for cardiovascular diseases that faces challenges during clinical trials is methotrexate. As indicated by the study conducted by Moreira et al (48), methotrexate demonstrates no disparity in the duration of a 6-minute walk before and after treatment. It also exhibits no impact on the levels of CRP, nor does it affect the incidence of MACE when compared to a placebo. Moreover, the same author’s additional study (49) reveals that methotrexate has no effect on the release of creatine kinase during the initial 72 hours, no discrepancy in CRP levels, a significantly poorer left ventricular ejection fraction (LVEF) in the methotrexate group at 3 months, and no impact on the incidence of MACE. Conversely, in Ridker et al’s (50) study, the trial was terminated prematurely due to the absence of any benefits. No difference was observed in the incidence of MACE or in the levels of inflammatory markers (white blood cell count, CRP, interleukin-1β, interleukin-6) at 8 months. However, an increased occurrence of leukopenia and non-basal cell skin cancer was noted.
The final example in the realm of cardiovascular medicine is the drug known as cyclosporine. Since its introduction into clinical practice in the late 1970s (51), the discovery and utilization of cyclosporine has had a significant impact on the advancement of transplant medicine (52,53). While it has shown improvements in the rates of acute rejection and early graft survival, the evidence regarding long-term survival of renal allografts is less convincing (54). The identification of acute reversible nephrotoxicity and nephrotoxicity in nonrenal transplants has subsequently led to the widely accepted notion that there is also a chronic and more irreversible component to this drug (55). Consequently, there has been a strong interest in developing protocols that aim to minimize or even eliminate the use of calcineurin inhibitors altogether (56). Despite these considerations, cyclosporine has been repurposed for various other diseases, including cardiovascular diseases (57,58). In two separate trials involving patients with ST-segment elevation myocardial infarction (STEMI), cyclosporine failed to demonstrate any beneficial effects on ST-segment resolution, left ventricular remodeling, or the incidence of cardiovascular events during the respective trial periods of 6 months and 1 year (57,58). Moreover, the use of cyclosporine in cardiovascular medicine has been associated with certain side effects such as hypertension, hyperlipidemia, and diabetes mellitus (59). These side effects may contribute to the high cardiovascular morbidity in renal transplant patients and the development of chronic transplant nephropathy (60). In conclusion, while cyclosporine has revolutionized transplant medicine, its use in cardiovascular medicine comes with potential side effects that must be carefully monitored and managed to ensure patient safety and optimal outcomes (61).
Challenges in cancer endeavor
In the field of hematological malignancies, despite the introduction of drug repurposing, which presents new possibilities for innovative treatments, achieving complete remission remains an arduous task (62). Clinical trials that explore the repurposing of drugs in oncology have exhibited varying levels of success, with certain limitations such as limited sample sizes and participant heterogeneity (63). An illustration of repurposing challenges in multiple clinical trials has come to light. One such instance involves the repurposing of bortezomib to target hematological malignancies. However, this drug proved to be ineffective as a stand-alone treatment for other malignancies, including acute myelogenous leukemia (AML) (62). Another example that showcases the act of appropriating preexisting resources for alternative purposes pertains to the potential drawbacks that arise when repurposing the chemical compound valproic acid (VPA) for various medical applications. These disadvantages, such as its lack of improvement in clinical outcomes for patients with myelodysplastic syndrome (MDS) or elderly patients with AML in a randomized phase II study of low-dose decitabine with VPA, have been observed (64). Additionally, another study found that VPA did not have an impact on the objective response rate or overall survival (65). Furthermore, a randomized phase III study examining the safety and efficacy of combining VPA and all-trans retinoic acid (ATRA) with induction therapy (idarubicin and cytarabine) for the treatment of elderly AML patients was terminated prematurely due to the absence of clinical improvement in the VPA group compared to standard treatment (66). This lack of improvement was further compounded by hematologic toxicity and higher mortality rates associated with VPA (67). All of these clinical studies shed light on the limitations and challenges inherent in using VPA in contexts other than its original intention. However, there is promising potential for computational drug repurposing to forecast the effectiveness of repurposed medications in phase III clinical trials, thereby streamlining the process (68).
Challenges in diabetes endeavor
Drug repurposing trials in the field of diabetes have encountered numerous hurdles and instances of failure. A particular study examined the inability of prominent pharmaceutical companies to develop novel treatments for type 2 diabetes (T2D) despite significant investments in traditional drug development pipelines (69). Another scholarly article highlighted the challenges faced by potentially effective medications during the advanced stages of clinical trials for diabetes management (70). Researchers in the same field are presently attempting to repurpose antidiabetic medications with the aim of addressing dementia, characterized by the prevalence of insulin sensitizers and insulin substrates. Despite the initial appearance of promise and the potential for success, none of the clinical trials conducted thus far have achieved the desired outcome of mitigating the cognitive deterioration associated with late-onset dementia (71).
In recent years, there has been a significant focus on the repurposing of antidiabetic drugs for the purpose of weight control, alongside the use of other medications. The use of antidiabetic drugs, such as metformin, glyburide, and SGLT-2 inhibitors, has shown promising results in terms of promoting weight loss, which has garnered considerable attention (72). Additionally, disulfiram, a medication commonly prescribed for chronic alcoholism, has demonstrated a significant capability to counteract obesity in rats (73). Although the specific mechanisms by which these drugs facilitate weight loss are still being investigated, their potential as treatments for obesity should not be underestimated. It is important to note that despite the positive effects of these repurposed drugs for obesity, there is also a focus on the repurposing of antidiabetic drugs such as semaglutide and tirzepatide, which were initially developed to address the challenges associated with T2D (74). These drugs can be traced back to a peptide that was discovered in the venom of the Gila monster and have the ability to mimic the actions of a naturally occurring hormone that is released after food consumption. As a result, they have shown promising results in terms of inducing weight loss. However, it is essential to acknowledge that these drugs do come with their fair share of adverse effects, particularly in the gastrointestinal realm. Potential side effects such as nausea, vomiting, diarrhea, and constipation have been reported with their use. Therefore, it is crucial to strike a careful balance between the beneficial effects and the potential adverse effects when considering the use of these drugs for the treatment of obesity.
Potential risk of repurposed antibiotics
One of the foremost concerns that need careful study is the repurposing of antibiotics for noninfectious disorders, some antibiotics of which are listed in Table 1. In this context, it is crucial to assess both the efficacy of these repurposed drugs and their potential to exacerbate the problem of multidrug resistance (75). In a study conducted by Wang et al (76), it was shown that some non-antibiotic medications, when present at levels that are both clinically and ecologically relevant, had a notable impact on the dissemination of antibiotic resistance. This effect was shown through the absorption of exogenous antibiotic resistance genes (ARGs) (77,78), due to the fact that the administration of non-antibiotic substances has the potential to increase the likelihood of the development of multidrug resistance at the clinical level (79). It is plausible to suggest that the utilization of repurposed drugs could exacerbate this issue even further. Therefore, the present work strives to clarify this threat in the subsequent section. The phenomenon of antibiotic resistance has been steadily increasing over the past few decades, with no new categories of antibiotics or viable alternatives receiving clinical approval within the last three decades (75,80).
Antibiotic | Repurposed for | Microbes resistant to this antibiotic | |
---|---|---|---|
1 | Levofloxacin |
- Lung cancer - Alzheimer’s disease - Anti-amyloidogenic |
- Cutibacterium avidum (82) - Stenotrophomonas maltophilia (83,84) - Haemophillus, Streptococcus, Corynebacterium, Staphylococcus, and Bordetella (85) - Helicobacter pylori (86) - Acinetobacter baumannii (87) - Acinetobacter spp. and Escherichia coli (88) |
2 | Benzylpenicillin | Alzheimer’s disease |
- All of the strains of nonfermenting gram-negative bacteria (NGNB) exhibited resistance to benzylpenicillin. Pseudomonas cepacia and P. stutzeri were identified as the species displaying the highest levels of resistance (89). . - Pseudomonas aeruginosa (90) - Indole-positive Proteus strains are uniformly resistant to benzylpenicillin (91). |
3 | Doxycycline | Treatment of malaria |
- A range of anaerobic bacteria, such as Bac teroides fragilis, exhibit resistance to attainable blood levels of doxycycline (92). - In the oral cavity, the prevailing type of antibiotic-resistant bacteria discovered were gram-positive cocci (93). - All isolated gram-negative strains, such as E. coli, E. fergusonii, and Proteus mirabilis, exhibited resistance to doxycycline (94). |
4 | Azithromycin |
- COVID-19 pandemic - Treatment of malaria |
25 different strains of Legionella pneumophila exhibited resistance to the antimicrobial drug azithromycin. |
There is an ongoing endeavor to discover novel drugs or alternative approaches to combat bacterial infections, but unfortunately, the rate at which bacterial resistance develops surpasses the pace of these endeavors (81).
While the notion of repurposing drugs may appear rational and a scientific approach to addressing previously incurable ailments such as Alzheimer’s, cancer, and Parkinson’s, the repurposing of antibiotic medications has generated concerns regarding their use in treating such diseases, particularly in light of the widespread prevalence of AMR that has wreaked havoc in our contemporary era. AMR is well-known for its devastating impact, with over 700,000 deaths occurring globally as a result, and projections indicating that this number could escalate to approximately 10 million people worldwide by the year 2050 (95). A number of research studies have delved into the utilization of antibiotics for the treatment of conditions other than bacterial infections, attempting to repurpose these medications (96-98). However, these studies have failed to adequately acknowledge the grave peril associated with employing antibiotics for repurposing, namely the progression of AMR (as depicted in Fig. 1).
The antibiotic levofloxacin has recently been proposed as a potential repurposed medication for Alzheimer’s disease (AD) (98). However, several other studies examining the effects of this antibiotic on Alzheimer’s patients have been disregarded. One of these studies involves a case report that observed an increase in seizures in an AD patient who was administered a dose of 500 mg/day of levofloxacin (99). Additionally, research on the gut microbiome of Alzheimer’s patients has indicated that Helicobacter pylori infection may serve as a trigger for the release of inflammatory mediators in AD patients (104). It is worth noting that H. pylori is known for its resistance to levofloxacin (Tab. 1) (105). The primary concern with repurposed antibiotics lies in their dosage, duration of use, and their impact on AMR. Antibiotics are typically administered at doses significantly higher than non-antibiotic drugs (27). In fact, a concentration of 400 mg of minocycline was found to be ineffective in delaying the progression of cognitive or functional impairment in patients with mild AD over a 2-year period (106). Despite their potential as candidates in laboratory settings, the efficacy of these antibiotics in vivo is not guaranteed. Moreover, long-term exposure to antibiotics, whether at clinical or sub-stoichiometric doses, can lead to the development of drug resistance among gut microbes. Chronic administration of these antibiotics is necessary for the treatment or management of AD. Consequently, the chronic use of antibiotics at clinical doses may result in antibiotic resistance, making it challenging to treat common infections. Furthermore, prolonged antibiotic use may increase a patient’s susceptibility to infections. If any antibiotics demonstrate potential as anti-Alzheimer’s agents in either laboratory or clinical studies, it is imperative to assess their long-term consequences.
There has been a lack of comprehensive research aimed at comprehending the prolonged implications of utilizing repurposed antibiotics to enhance AMR. However, existing data have demonstrated a direct correlation between the consumption of antibiotics and the emergence of AMR (107). Consequently, an escalation of multidrug resistance may occur as the consumption of these repurposed antibiotics increases. For example, doxycycline is an antibiotic that has been repurposed for the treatment of malaria (Tab. 1). While there is no documented evidence of increased AMR due to the use of doxycycline for malaria prophylaxis, reports have indicated the development of doxycycline resistance and coresistance to other antibiotics when used to treat other diseases. A study revealed that doxycycline-induced stress led to the emergence of coresistance to colistin, which is considered a last-resort antibiotic for extensively drug-resistant bacteria (108). This study shed light on a potential mechanism of doxycycline-selected resistance and coresistance in Vibrio cholerae, emphasizing the need for stringent regulations regarding the indiscriminate use of antibiotics. Another aspect to consider is the unrestricted sale of these antibiotics without prescription, particularly in developing countries such as India, which is one of the largest consumers of antibiotics (109). Consequently, the controlled usage of these repurposed antibiotics may be compromised. Prolonged use of repurposed antibiotics could exert selective pressure, resulting in the survival of multidrug-resistant and extremely drug-resistant bacteria. In the absence of a competitive environment, these drug-resistant strains may thrive more successfully, leading to the elimination of phenotypically sensitive strains, as illustrated in Figure 3 (110).
During the COVID-19 pandemic, health professionals turned to the administration of azithromycin alone or in combination with drugs like hydroxychloroquine. This repurposing of an antibiotic without any evidence of its antiviral properties became a highly controversial practice. However, its utilization continued to increase with the successive waves of COVID-19. The future implications of such unregulated and unscientific use of antibiotics remain uncertain. The consequences of this practice on AMR will further exacerbate the situation (111). In addition to the repurposing of azithromycin in the context of the COVID-19 pandemic, numerous studies have revealed its antimalarial properties. The eradication of Plasmodium falciparum parasites is accomplished through two distinct mechanisms: delayed death by means of inhibiting the apicoplast ribosomes and rapid elimination throughout the blood stage development (112). A trial carried out in Burkina Faso discovered that a solitary oral dose of azithromycin did not result in a reduction in malaria positivity; however, it did alleviate caregiver-reported fever as an adverse event (113). Furthermore, an additional study determined that the supplementation of azithromycin to seasonal malaria chemoprevention did not yield improved nutritional outcomes among children (114). Furthermore, novel derivatives of azithromycin that exhibit swift action have been developed, manifesting exceptional antimalarial activity in both in vitro and in vivo settings, while employing a distinct mode of action compared to the more gradual acting azithromycin (115). Although azithromycin has indeed exhibited potential as an antimalarial agent, further investigation is necessary to optimize its efficacy and comprehend its mechanisms of action. Considering the positive correlation between extensive antibiotic usage and the worsening of the antibiotic resistance crisis in the present era, it is imperative to enhance AMR stewardship at an international level in order to mitigate the impact of antibiotic use on the menace of antibiotic resistance. Particular emphasis should be placed on comprehending the ramifications of antibiotic repurposing.
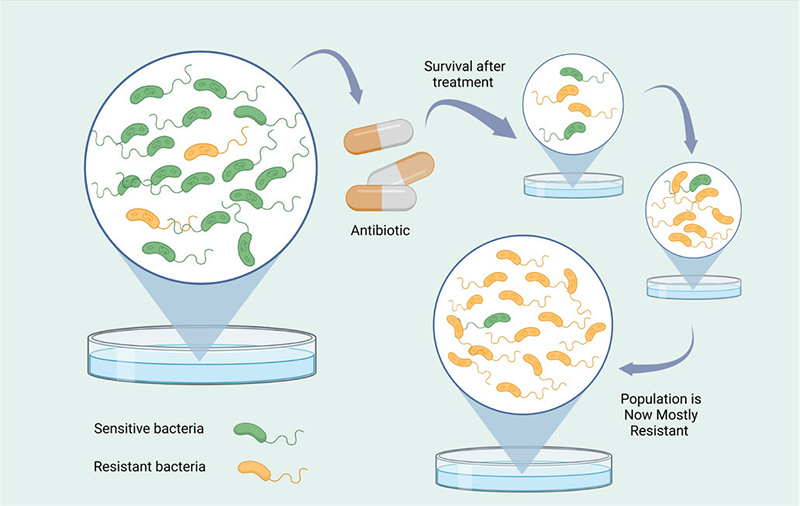
FIGURE 3 - Progression of antimicrobial resistance. Antimicrobial resistance may arise as a consequence of the improper utilization of antibiotics or their employment for indications other than the treatment of bacterial infections (100). This resistance can manifest through the transmission of genes associated with antimicrobial resistance when bacteria are subjected to prolonged exposure to low levels of antibiotics (101). Such a situation can result in the emergence of bacteria that are resistant to multiple drugs, thereby impeding the effective management of bacterial infections and presenting a significant public health challenge (102). To effectively address antimicrobial resistance, it is imperative to advocate for the responsible and prudent utilization of antibiotics, ensuring their administration solely when warranted and in accordance with appropriate dosages and durations (103). This diagram elucidates the manner in which the inappropriate employment of antibiotics, whether in terms of sublethal dosages or durations, leads to the dissemination of resistance genes within bacterial populations, consequently giving rise to an entirely resistant population.
There is a profound necessity to explore alternatives to antibiotics in the context of repurposing, especially those that have already demonstrated resistance. The successful repurposing of non-antibiotic drugs for cancer treatment has been documented (116,117) and a similar approach can be adopted for other diseases as well. While the repurposed antibiotics may circumvent clinical trials, the absence of substantial studies addressing the grave issue of AMR may prove to be catastrophic for the already advancing AMR against most antibiotics. The role of AMR in exacerbating challenges in the management of nosocomial infections and the resulting mortality due to multidrug resistance should not be underestimated, particularly in an era where the efficacy of most antibiotics is waning and treatment regimens rely on last-resort antibiotics (118). It is imperative to find a solution that does not further burden the existing load of AMR. Therefore, we propose that clinicians and researchers raise awareness about the rampant problem of AMR and its consequences, while discouraging the use of antibiotics in drug repurposing.
After considering the knowledge mentioned above, one could potentially inquire whether the practice of repurposing antibiotics to address noninfectious ailments could potentially elevate the likelihood of AMR. Furthermore, one may also wonder about the implications of reusing antibiotics that were originally approved for the treatment of one specific infection in the context of treating a different infection.
In previous times, newer antibiotics were consistently being surpassed by more recent ones, which were devised to circumvent the most recent resistance mechanism discovered in clinical isolates. Nevertheless, numerous companies have abandoned initiatives aimed at developing novel anti-infectives due to the time and expense associated with clinical trials. The insufficient availability of new drugs has provided a compelling impetus to repurpose older antibiotics for treating multidrug-resistant strains or to combine them with agents capable of targeting multiple distinct pathways within the pathogen or activating the resistance pathways of host cells. This approach is anticipated to result in heightened effectiveness and reduce the likelihood of resistance emergence (119).
Reverting to previous antibiotics can, under certain circumstances, overcome antibiotic resistance. For instance, strains of tuberculosis that are resistant to the drug combinations currently in use can be found in all regions of the world. One effective tuberculosis medication, known as isoniazid, hinders the enzyme InhA, thereby obstructing the synthesis of the mycolic acids that constitute the cell wall of the mycobacterium. The resistance to isoniazid, which is a prodrug, largely arises from mutations in the bacterial enzymes that are necessary for its activation. Recently, it has been discovered that an older antibiotic derived from natural sources, called pyridomycin and first identified in the 1950s, directly inhibits InhA. Consequently, it can be employed to treat infections caused by drug-resistant strains of tuberculosis that survive due to their inability to activate isoniazid (120). The comprehension of this mechanism of action may also promote the utilization of pyridomycin in conjunction with other medications (121). However, there are several difficulties in repurposing drugs for infectious diseases, and the subsequent sections will outline some of the primary challenges that arise in this domain.
The complexity of microorganisms and the limited understanding of the complicated interactions between pathogens and their hosts
Identifying appropriate therapeutic targets for anti-infective medications is a challenging endeavor due to the complex nature of infection processes and the intricate interplay between hosts and pathogens (122). Pathogens, encompassing viruses, bacteria, protozoa, and nematodes, acquire significant genetic diversity through mutation and recombination, resulting in the emergence of drug-resistant mutants (123). Consequently, the development of drugs that can effectively target all viral variations becomes arduous. Hence, it is imperative to consistently exert efforts to stay ahead of evolving resistance mechanisms (124). Furthermore, certain infections, including Neisseria spp. or Plasmodium spp., possess the capability to undergo antigenic variation, wherein they modify the surface proteins on their cells to evade detection and attack by the host organism’s immune system. This ongoing process of adaptation poses challenges in identifying consistent therapeutic targets and potential candidates for vaccination (125). Additionally, several pathogens like Trypanosoma cruzi, Mycobacterium tuberculosis, and herpes viruses can establish latent or chronic infections, wherein they remain inactive within host cells and successfully evade immune detection (126,127). Effectively managing such infections requires medications that can specifically target the pathogen during both its active and latent stages. Moreover, certain bacteria such as Pseudomonas aeruginosa, Acinetobacter baumannii, and Staphylococcus aureus can form biofilms, which augment their resistance to drugs and impede drug accessibility, thereby making the eradication of infections more formidable (75,128).
Lack of efficient animal models
Developing animal models that accurately replicate human infections is essential for preclinical testing. However, creating suitable models for specific infectious diseases can pose challenges due to the intricate interactions between hosts and pathogens that are difficult to accurately simulate (129). The absence of appropriate predictive models can hinder the progress of drug development, as it becomes arduous to comprehend the disease mechanisms and evaluate the effectiveness of potential medications (130). An example is the complexity involved in modeling diseases like COVID-19 that necessitates the consideration of various factors such as stochasticity, evolving transmission dynamics, and spatial configurations (131).
A significant advancement in the field of antimalarial drug research and development was the establishment of a NOD-SCID mouse model for P. falciparum infection, which proved valuable for testing drug efficacy (132). Moreover, the utilization of organoid cultures derived from stem cells holds significant promise in providing valuable insights into infectious diseases by effectively mimicking in vivo disease characteristics and enhancing the comprehension of host-microbe interactions (133,134). In addition, the integration of sophisticated mathematical models such as the SEIR model, incorporating multiple variables, can greatly assist in forecasting disease dissemination, particularly for infections characterized by prolonged incubation periods and asymptomatic carriers (135). The optimization of the modeling process through the formulation of well-defined research questions, implementation of quality assurance measures, and meticulous reporting practices can substantially elevate the relevance and comprehensibility of findings, thereby playing a pivotal role in improving decision-making processes related to disease management (136).
On the other hand, epidemiological models, such as agent-based simulation models and compartmental models, play a crucial role in understanding disease spread dynamics and evaluating intervention strategies (137-139). These models often involve making assumptions that may limit their scope, such as focusing on single-strain and single-vector scenarios, simplistic human behavior modeling, and ignoring data quality evaluation (140). To improve the reliability of these models, it is essential to consider factors like information quality, human behavior, multi-vector, and multi-strain scenarios, as they significantly impact the model outcomes without significantly increasing computational costs (141). Collaborative efforts between researchers and modelers are crucial to enhancing the accuracy of predictions and optimizing models for effective public health decision-making and for repurposing antibiotics.
Elevated likelihood of clinical failure
Clinical trials for anti-infective medications frequently encounter significant attrition rates as a result of inadequate effectiveness, apprehensions regarding safety, or difficulties in the recruitment of patients (142). Additionally, the execution of clinical trials and the subsequent monitoring in resource-constrained environments, along with the absence of suitable surrogate endpoints for infectious diseases, collectively contribute to the elevated levels of unsuccessful outcomes (143).
In conclusion, while there is potential in repurposing drugs to overcome antibiotic resistance in infectious diseases, several difficulties arise in this domain. The complexity of microorganisms and the intricate interactions between pathogens and their hosts pose challenges in identifying suitable therapeutic targets. Pathogens’ ability to evolve, undergo antigenic variation, establish latent or chronic infections, and form biofilms further complicate the development of effective medications. Addressing these challenges will require continued research and collaboration to develop novel strategies and therapies to combat drug-resistant infections.
Conclusion
Drug repurposing is a strategic initiative that involves the exploration and identification of fresh therapeutic applications for pharmaceuticals that already exist in the market. This approach has proven to be beneficial due to its cost-effectiveness and ability to save time in the drug discovery process. However, it is important to consider various factors before fully embracing this strategy. One such factor is the fact that numerous repurposed drugs have failed to achieve their intended targets during clinical trials, leading to concerns about the effectiveness of this approach. Additionally, there is a growing apprehension about the potential increase in the risk of multidrug resistance, which further emphasizes the need for caution when repurposing drugs.
The development of repurposed medications requires a high level of creativity and innovation in designing the development program. This is because each case presents a unique set of circumstances and evidence that must be thoroughly evaluated. Therefore, it is crucial to approach each drug repurposing project with a comprehensive understanding of the ethical and pharmaceutical considerations involved. Furthermore, it is imperative that repurposed drugs undergo rigorous clinical trials before they can be approved for use. This ensures that the safety and efficacy of these drugs are thoroughly evaluated, and any potential risks and side effects are identified and mitigated. In conclusion, the process of selecting a repurposed drug should be guided by a range of ethical and pharmaceutical considerations. It is important to acknowledge the limitations and challenges associated with drug repurposing, such as the potential failure to reach therapeutic targets and the risk of multidrug resistance. By prioritizing the application of creativity and innovation in the development program, as well as ensuring the completion of rigorous clinical trials, we can maximize the potential benefits of repurposed drugs while minimizing the associated risks.
Disclosures
Conflicts of interest: The authors declare no conflict of interest.
Funding: This research received no external funding.
Data availability statement: The data presented in this study are available on request from the corresponding author.
Author contributions: Conceptualization, I.Y.N. and M.M.A.; software, D.K.A. and M.M.D.; validation, I.Y.N. and A.K.A.; formal analysis, D.K.A. and M.M.A.; investigation, A.K.A. and M.M.D.; resources, I.Y.N., M.M.D., MJ.A.S., and A.K.A.; data curation, D.K.A. and M.M.A.; writing—original draft preparation, M.M.A. and I.Y.N.; writing—review and editing, M.M.A., MJ.A.S., and D.K.A.; visualization, I.Y.N., MJ.A.S., and A.K.A.; supervision, M.M.A. and I.Y.N.; project administration, I.Y.N. All authors have read and agreed to the published version of the manuscript.
References
- 1. Agnihotri Jaya, R Sunanda, Patil et al. “Drug repurposing: A futuristic approach in drug discovery”. Journal of pharmaceutical and biological sciences, vol. 11, no. 1, 66-69, 2023.
- 2. Hyeong-Min L, Yuna K. Drug repurposing is a new opportunity for developing drugs against neuropsychiatric disorders. Schizophr Res Treatment. 2016;2016:6378137.
- 3. Meera M, Sekar S, Mahatao R. A novel approach for drug discovery-drug repurposing. Natl J Physiol Pharm Pharmacol. 2022;12(5):546-551.
- 4. Iacopetta D. Special issue on “anticancer drugs activity and underlying mechanisms”. Appl Sci (Basel). 2021;11(17):8169. CrossRef
- 5. Pardo-Yules B, Gallego-Durán R, Eslam M, et al. Thalidomide with peginterferon alfa-2b and ribavirin in the treatment of non-responders genotype 1 chronic hepatitis C patients: proof of concept. Rev Esp Enferm Dig. 2011;103(12):619-625. CrossRef PubMed
- 6. Jesus SM, Santana RS, Leite SN. The organization, weaknesses, and challenges of the control of thalidomide in Brazil: a review. PLoS Negl Trop Dis. 2020;14(8):e0008329. CrossRef PubMed
- 7. Duarte D, Vale N. Antidepressant drug sertraline against human cancer cells. Biomolecules. 2022;12(10):1513. CrossRef PubMed
- 8. Rehan M, Ahmed F, Howladar SM, et al. A computational approach identified andrographolide as a potential drug for suppressing Covid-19-induced cytokine storm. Front Immunol. 2021;12:648250.
- 9. Talib Jawad Kadhim, Omer Abd Alkareem Khalf. A review search of sildenafil uses in human and in the veterinary medicine. AIP Conf Proc. 2023;2475:100010.
- 10. Al Ibrahim AH, Ghallab KQ, Alhumaid FI, et al. A systematic review of sildenafil mortality through the years. Cureus. 2022;14(12):e32179.
- 11. Hussein MA, Salah El-Din MM, Saleh EM, et al. Sildenafil (VIAGRATM): a promising anticancer drug against certain human cancer cell lines. Asian J Chem. 2021;33(6):1420-1424. CrossRef
- 12. Goldstein I, Burnett AL, Rosen RC, et al. The serendipitous story of sildenafil: an unexpected oral therapy for erectile dysfunction. Sex Med Rev. 2019;7(1):115-128. CrossRef PubMed
- 13. Ala M, Jafari RM, Dehpour AR. Sildenafil beyond erectile dysfunction and pulmonary arterial hypertension: thinking about new indications. Fundam Clin Pharmacol. 2021;35(2):235-259.
- 14. Mpoeo, A Baa, Ky. Hooe peaaee capx eapc (oop). apaoa pecpa eapcex cpec, 2023;12(1):182-190. CrossRef
- 15. Krishnamurthy N, Grimshaw AA, Axson SA, Choe SH, Miller JE. Drug repurposing: a systematic review on root causes, barriers and facilitators. BMC Health Serv Res. 2022;22(1):1-17. CrossRef PubMed
- 16. Trivedi J, Mohan M, Byrareddy SN. Drug repurposing approaches to combating viral infections. J Clin Med. 2020;9(11):3777. CrossRef PubMed
- 17. Ekeomodi CC, Obetta KI, Okolocha ML, et al. Computational approaches in drug repurposing. In Rudrapal M, editor, Drug repurposing – advances, scopes and opportunities in drug discovery. IntechOpen; 2023.
- 18. Tuerkova A, Zdrazil B. A ligand-based computational drug repurposing pipeline using KNIME and Programmatic Data Access: case studies for rare diseases and COVID-19. J Cheminform. 2020;12(1):71. CrossRef PubMed
- 19. Jian Hong Gan, Ji Xiang Liu, Yang Liu, et al. DrugRep: an automatic virtual screening server for drug repurposing. Acta Pharmacol Sinica. 2023;44(4):888-896.
- 20. Sadegh S, Skelton J, Anastasi E, et al. NeDRex – an integrative and interactive network medicine platform for drug repurposing. In RExPO22, The 1st International Conference on Drug Repurposing, Maastricht; 2022. CrossRef
- 21. Parmar G, Chudasama JM, Shah A, Patel A. In silico pharmacology and drug repurposing approaches. In Rudrapal M, Khan J, eds, CADD and informatics in drug discovery. Springer; 2023: 253-281. CrossRef
- 22. Khan S, Agnihotri J, Patil S, Khan N. Drug repurposing: a futuristic approach in drug discovery. J Pharm Biol Sci. 2023;11(1):66-69. CrossRef
- 23. Geest R, Nijholt D. Hollander W. Clinical Development in Drug Repurposing. In RExPO22, The 1st International Conference on Drug Repurposing, Maastricht; ScienceOpen, August 2022.
- 24. Tan GSQ, Sloan EK, Lambert P, Kirkpatrick CMJ, Ilomäki J. Drug repurposing using real-world data. Drug Discov Today. 2023;28(1):103422. CrossRef PubMed
- 25. Juárez-López D, Schcolnik-Cabrera A. Drug repurposing: considerations to surpass while re-directing old compounds for new treatments. Arch Med Res. 2021;52(3):243-251. CrossRef PubMed
- 26. Selvaraj N, Swaroop AK, Nidamanuri BSS, Kumar RR, Natarajan J, Selvaraj J. Network-based drug repurposing: a critical review. Curr Drug Res Rev. 2022;14(2):116-131. CrossRef PubMed
- 27. Farha MA, Brown ED. Drug repurposing for antimicrobial discovery. Nat Microbiol. 2019;4(4):565-577. CrossRef PubMed
- 28. Fetro C, Scherman D. Drug repurposing in rare diseases: myths and reality. Therapie. 2020;75(2):157-160. CrossRef PubMed
- 29. Aggarwal NN, Sindhoor SM, Naveen NR, Gowthami B, Biju P. Drug reprofiling: a prospective approach to battle chronic ailments. J Health Allied Sci 2023;14:38-46.
- 30. Annabell C, David R, Greaves R. Drug repurposing in cardiovascular inflammation: successes, failures, and future opportunities. Front Pharmacol. 2022;13:1046406.
- 31. Ridker PM, Everett BM, Thuren T, et al. Antiinflammatory therapy with canakinumab for atherosclerotic disease. N Engl J Med. 2017;377(12): 1119-1131.
- 32. Dhimolea E. Canakinumab. MAbs. 2010;2(1):3-13. CrossRef PubMed
- 33. Miller J. FDA snubs Novartis bid to repurpose inflammation drug for heart attacks, October 2018. Online.
- 34. Davis JC Jr, Heijde D, Braun J, et al. Recombinant human tumor necrosis factor receptor (etanercept) for treating ankylosing spondylitis: a randomized, controlled trial. Arthritis Rheumatol. 2003;48(11):3230-3236.
- 35. Gatti J, Lindstrom JA, Beitz J. Reconsideration of 2008 decision: Food and Drug Administration approval of etanercept for systemic treatment of moderate to severe pediatric psoriasis. Pediatr Dermatol. 2018;35(5):688-689. CrossRef PubMed
- 36. Nathan Mewton, François Roubille, Didier Bresson et al. “Effect of colchicine on myocardial injury in acute myocardial infarction”. Circulation, vol. 144, no. 11, 859–869, 2021.
- 37. Shah B, Pillinger M, Zhong H, et al. Effects of acute colchicine administration prior to percutaneous coronary intervention: COLCHICINE-PCI randomized trial. Circ Cardiovasc Interv. 2020;13(4):e008717. CrossRef PubMed
- 38. Tardif J-C, Kouz S, Waters DD, et al. Efficacy and safety of low-dose colchicine after myocardial infarction. N Engl J Med. 2019;381(26):2497-2505.
- 39. Tong DC, Quinn S, Nasis A, et al. Colchicine in patients with acute coronary syndrome: the Australian COPS randomized clinical trial. Circulation. 2020;142(20):1890-1900.
- 40. Mewton N, Roubille F, Bresson D, et al. Effect of colchicine on myocardial injury in acute myocardial infarction. Circulation. 2021;144(11):859-869. CrossRef PubMed
- 41. Spada A, Emami J, Sanaee F, et al. Design and evaluation of albumin nanoparticles for the delivery of a novel β-tubulin polymerization inhibitor. J Pharm Pharm Sci. 2021;24:344-362. CrossRef PubMed
- 42. Kaddoura M, AlIbrahim M, Hijazi G, et al. Covid-19 therapeutic options under investigation. Vol 11. In Uckun FMM. Frontiers in pharmacology; 2020.
- 43. Ge P, Fu Y, Qi S, et al. Colchicine for prevention of post-operative atrial fibrillation: meta-analysis of randomized controlled trials. Vol 9. Front Cardiovasc Med. 2022;9:1032116.
- 44. Akrami M, Izadpanah P, Bazrafshan M, et al. Effects of colchicine on major adverse cardiac events in next 6-month period after acute coronary syndrome occurrence; a randomized placebo-control trial. BMC Cardiovasc Disord. 2021;21(1):583. CrossRef PubMed
- 45. Meyer-Lindemann U, Mauersberger C, Schmidt A, et al. Colchicine impacts leukocyte trafficking in atherosclerosis and reduces vascular inflammation. Front Immunol. 2022;13:898690.
- 46. Huang W, Wang Y, Tian W, et al. Biosynthesis investigations of terpenoid, alkaloid, and flavonoid antimicrobial agents derived from medicinal plants. Antibiotics (Basel). 2022;11(10):1380. CrossRef PubMed
- 47. Zhang F-S, He Q-Z, Qin CH, Little PJ, Weng JP, Xu SW. Therapeutic potential of colchicine in cardiovascular medicine: a pharmacological review. Acta Pharmacol Sin. 2022;43(9):2173-2190. CrossRef PubMed
- 48. Moreira DM, Vieira JL, Gottschall CA. The effects of METhotrexate therapy on the physical capacity of patients with ISchemic heart failure: a randomized double-blind, placebo-controlled trial (METIS trial). J Card Fail. 2009;15(10):828-834. CrossRef PubMed
- 49. Moreira DM, Lueneberg ME, da Silva RL, Fattah T, Gottschall CAM. MethotrexaTE THerapy in ST-segment elevation mYocardial infarctionS: a randomized double-blind, placebo-controlled trial (TETHYS Trial). J Cardiovasc Pharmacol Ther. 2017;22(6):538-545. CrossRef PubMed
- 50. Ridker PM, Everett BM, Pradhan A, et al; CIRT Investigators. Low-dose methotrexate for the prevention of atherosclerotic events. N Engl J Med. 2019;380(8):752-762. CrossRef PubMed
- 51. Borel JF. Comparative study of in vitro and in vivo drug effects on cell-mediated cytotoxicity. Immunology. 1976;31(4):631-641. PubMed
- 52. Tedesco D, Haragsim L. Cyclosporine: a review. J Transplant. 2012;2012:230386.
- 53. Lim SW, Doh KC, Jin L, et al. Oral administration of ginseng ameliorates cyclosporine-induced pancreatic injury in an experimental mouse model. PLoS One. 2013;8(8):e72685. CrossRef PubMed
- 54. Kern G, Mair SM, Noppert SJ, et al. Tacrolimus increases Nox4 expression in human renal fibroblasts and induces fibrosis-related genes by aberrant TGF-beta receptor signalling. PLoS One. 2014;9(5):e96377. CrossRef PubMed
- 55. Thorat A, Chou H-S, Lee C-F, et al. Effects of converting tacrolimus formulation from twice-daily to once-daily in liver transplantation recipients. BioMed Res Int. 2014;2014:265658.
- 56. Abdelkader M, Hilal M, Torky AR, Elsayed H, Allam W. Experimental study of renal toxicity of cyclosporine and the ameliorative effect of N-acetylcysteine in albino rat. Ain Shams J Forensic Med Clin Toxicol. 2021;37(2):8-15. CrossRef
- 57. Cung T-T, Morel O, Cayla G, et al. Cyclosporine before PCI in patients with acute myocardial infarction. N Engl J Med. 2015;373(11):1021-1031. CrossRef PubMed
- 58. Ottani F, Latini R, Staszewsky L, et al; CYCLE Investigators. Cyclosporine A in reperfused myocardial infarction: the multicenter, controlled, open-label CYCLE trial. J Am Coll Cardiol. 2016;67(4):365-374. CrossRef PubMed
- 59. Elgendy A, Alshawadfy E, Altaweel A, Elsaidi A. Cardiovascular and metabolic comorbidities of psoriasis. Dermatol Case Rep. 2016;1(1):1-9. CrossRef
- 60. Sakamoto H, Kurabayashi M. Cardiovascular effects of an immunosuppressive agent cyclosporin A. Int J Immunopathol Pharmacol. 2000;15(2):75-79. CrossRef PubMed
- 61. Grupper A, Shashar M, Bahry D, et al. Cyclosporine attenuates arginine transport, in human endothelial cells, through modulation of cationic amino acid transporter-1. Am J Nephrol. 2013;37(6):613-619. CrossRef PubMed
- 62. Michael AA, Balakrishnan P, Velusamy T. Drug repurposing for hematological malignancies. In Sobti RC, Lal SK, Goyal RK, eds. Drug repurposing for emerging infectious diseases and cancer, Singapore, Springer; 2023:217-252. CrossRef
- 63. Ioakeim-Skoufa I, Tobajas-Ramos N, Menditto E, et al. Drug repurposing in oncology: a systematic review of randomized controlled clinical trials. Cancers (Basel). 2023;15(11):2972. CrossRef PubMed
- 64. Issa J-P, Garcia-Manero G, Huang X, et al. Results of phase 2 randomized study of low-dose decitabine with or without valproic acid in patients with myelodysplastic syndrome and acute myelogenous leukemia. Cancer. 2015;121(4):556-561. CrossRef PubMed
- 65. Lübbert M, Grishina O, Schmoor C, et al. Results of the randomized phase II study decider (AMLSG 14-09) comparing decitabine (DAC) with or without valproic acid (VPA) and with or without all-trans retinoic acid (ATRA) add-on in newly diagnosed elderly non-fit AML patients. Blood. 2016;128(22):589. CrossRef
- 66. Tassara M, Döhner K, Brossart P, et al. Valproic acid in combination with all-trans retinoic acid and intensive therapy for acute myeloid leukemia in older patients. Blood. 2014;123(26):4027-4036. CrossRef PubMed
- 67. Wojcicki AV, Kadapakkam M, Frymoyer A, Lacayo N, Chae HD, Sakamoto KM. Repurposing drugs for acute myeloid leukemia: a worthy cause or a futile pursuit? Cancers (Basel). 2020;12(2):441. CrossRef PubMed
- 68. Zong N, Chowdhury S, Zhou S, et al. Artificial intelligence-based efficacy prediction of phase 3 clinical trial for repurposing heart failure therapies. medRxiv, 2023:2023.05.25.23290531.
- 69. Turner N, Zeng X-Y, Osborne B, Rogers S, Ye J-M. Repurposing drugs to target the diabetes epidemic. Trends Pharmacol Sci. 2016;37(5):379-389. CrossRef PubMed
- 70. Mandrup-Poulsen T. Perspective: testing failures. Nature. 2012;485(7398):S17. CrossRef PubMed
- 71. Mantik KEK, Kim S, Gu B, et al. Repositioning of anti-diabetic drugs against dementia: insight from molecular perspectives to clinical trials. Int J Mol Sci. 2023;24(14):11450. CrossRef PubMed
- 72. Pathak K, Pathak MP, Saikia R, et al. Therapeutic repurposing of antidiabetic drugs in diabetes-associated comorbidities. Curr Drug Ther. 2024;19(2):178-194.
- 73. Haddad F, Dokmak G, Bader M, Karaman R. A comprehensive review on weight loss associated with anti-diabetic medications. Life (Basel). 2023;13(4):1012. CrossRef PubMed
- 74. Gussow L. More questions than answers about injectable weight loss drugs. Emerg Med News. 2023;45(7):10. CrossRef
- 75. Natsheh IY, Elkhader MT, Al-Bakheit AA, et al. Inhibition of Acinetobacter baumannii biofilm formation using different treatments of silica nanoparticles. Antibiotics (Basel). 2023;12(9):1365. CrossRef PubMed
- 76. Wang Y, Lu J, Engelstädter J, et al. Non-antibiotic pharmaceuticals enhance the transmission of exogenous antibiotic resistance genes through bacterial transformation. ISME J. 2020;14(8):2179-2196. CrossRef PubMed
- 77. Stevenson C, Hall JP, Harrison E, Wood A, Brockhurst MA. Gene mobility promotes the spread of resistance in bacterial populations. ISME J. 2017;11(8):1930-1932. CrossRef PubMed
- 78. Gillings MR, Gaze WH, Pruden A, et al. Using the class 1 integron-integrase gene as a proxy for anthropogenic pollution. ISME J. 2015;9(6):1269-1279.
- 79. Chawla M, Verma J, Gupta R, Das B. Antibiotic potentiators against multidrug-resistant bacteria: discovery, development, and clinical relevance. Front Microbiol. 2022;13(887251):887251. CrossRef PubMed
- 80. Cunningham SA, Rodriguez C, Woerther P-L, et al. In vivo emergence of dual resistance to rifampin and levofloxacin in osteoarticular Cutibacterium avidum. Microbiol Spectr. 2023;11(4):e0368722.
- 81. Dulyayangkul P, Calvopiña K, Heesom KJ, Avison MB. Novel mechanisms of efflux-mediated levofloxacin resistance and reduced amikacin susceptibility in Stenotrophomonas maltophilia. Antimicrob Agents Chemother. 2020;65(1):e01284-20. CrossRef PubMed
- 82. Zając OM, Tyski S, Laudy AE. The contribution of efflux systems to levofloxacin resistance in Stenotrophomonas maltophilia clinical strains isolated in Warsaw, Poland. Biology (Basel). 2022;11(7):1044. CrossRef PubMed
- 83. Ramdhani D, Azizah SN, Kusuma SAF, Sediana D. Antibiotic resistance: evaluation of levofloxacin treatment in acute respiratory tract infections cases at the Tasikmalaya City Health Center, Indonesia. J Adv Pharm Technol Res. 2020;11(3):113-116. CrossRef PubMed
- 84. Trespalacios-Rangél AA, Otero W, Arévalo-Galvis A, Poutou-Piñales RA, Rimbara E, Graham DY. Surveillance of levofloxacin resistance in Helicobacter pylori isolates in Bogotá-Colombia (2009-2014). PLoS One. 2016;11(7):e0160007. CrossRef PubMed
- 85. Abdelaal AM, Mahmood SS. The role of efflux pump adeJ gene in levofloxacin resistance among A. baumannii. Syst Rev Pharm. 2020;11(10):1105-1110.
- 86. Asaduzzaman M, Hasan MZ, Khatun M, et al. Resistance pattern of levofloxacin against uropathogens causing urinary tract infection in selected areas of Dhaka city. Bangladesh J Biol Agric Healthc. 2018;8(4):74-81.
- 87. Iskhakova KhI. Antibiotic sensitivity of nonfermenting gram-negative bacteria. Antibiotiki i Khimioterapiia. 1988;33(11):823-827.
- 88. Rolinson GN. Bacterial resistance to penicillins and cephalosporins. Proc R Soc Lond, B. 1971;179(1057):403-410. CrossRef PubMed
- 89. Lachmajer-Lutoslawska M, Bobrowski M. Resistance to beta-lactam antibiotics Proteus strains. Acta Microbiol Pol A. 1975;8(3):141-149. PubMed
- 90. Chow AW, Patten V, Guze LB. Comparative susceptibility of anaerobic bacteria to minocycline, doxycycline, and tetracycline. Antimicrob Agents Chemother. 1975;7(1):46-49. CrossRef PubMed
- 91. Larsen T. Occurrence of doxycycline resistant bacteria in the oral cavity after local administration of doxycycline in patients with periodontal disease. Scand J Infect Dis. 1991;23(1):89-95. CrossRef PubMed
- 92. Eliopulos N, Alsina L, Diana L, Brandl S. Multiple antimicrobial resistance in Enterobacteriaceae isolated from a Sea Lion (Otaria flavescens) specimen from Isla de Lobos, Uruguay: a case report. Brazilian J Animal Environ Res. 2022;5(4):3477-3486. CrossRef
- 93. Belousoff MJ, Venugopal H, Wright A, et al. cryoEM-guided development of antibiotics for drug-resistant bacteria. ChemMedChem. 2019;14(5):527-531. CrossRef PubMed
- 94. Richardson LL. Alternating antibiotics render resistant bacteria beatable. PLOS Biol. 2015;13(4):e1002105.
- 95. O’neill J. Antimicrobial resistance: tackling a crisis for the health and wealth of nations. In Review on Antimicrobial Resistance. London: Wellcome Trust; 2014.
- 96. Song M, Wu H, Wu S, et al. Antibiotic drug levofloxacin inhibits proliferation and induces apoptosis of lung cancer cells through inducing mitochondrial dysfunction and oxidative damage. Biomed Pharmacother. 2016;84:1137-1143. CrossRef PubMed
- 97. Alsalahat I, Al-Majdoub ZM, Taha MO, et al. Inhibition of aggregation of amyloid-β through covalent modification with benzylpenicillin; potential relevance to Alzheimer’s disease. Biochem Biophys Rep. 2021;26:100943. CrossRef PubMed
- 98. Khan AN, Qureshi IA, Khan UK, Uversky VN, Khan RH. Inhibition and disruption of amyloid formation by the antibiotic levofloxacin: a new direction for antibiotics in an era of multi-drug resistance. Arch Biochem Biophys. 2021;714:109077. CrossRef PubMed
- 99. Lanckohr C, Bracht H. Antimicrobial stewardship. Curr Opin Crit Care. 2022;28(5):551-556. CrossRef PubMed
- 100. Rangapriya M, Lorance A, Varghese AM, Hanif A, Aruna S. Awareness and perception on antibiotics and antimicrobial resistance: a questionnaire based study. Int J Pharm Sci Rev Res. 2020;65(1):27-32. CrossRef
- 101. Xiang L, Akakuru OU, Xu C, Wu A. Harnessing the intriguing properties of magnetic nanoparticles to detect and treat bacterial infections. Magnetochemistry. 2021;7(8):112. CrossRef
- 102. Zhu D, Li Q, Shen Y, Zhang Q. Risk factors for quinolone-resistant Escherichia coli infection: a systematic review and meta-analysis. Antimicrob Resist Infect Control. 2020;9:11.
- 103. Bird SB, Orr PG, Mazzola JL, Brush DE, Boyer EW. Levofloxacin-related seizure activity in a patient with Alzheimer’s disease: assessment of potential risk factors. J Clin Psychopharmacol. 2005;25(3):287-288. CrossRef PubMed
- 104. Doulberis M, Kotronis G, Gialamprinou D, et al. Alzheimer’s disease and gastrointestinal microbiota; impact of Helicobacter pylori infection involvement. Int J Neurosci. 2021;131(3):289-301. CrossRef PubMed
- 105. Mehrotra T, Devi TB, Kumar S, et al. Antimicrobial resistance and virulence in Helicobacter pylori: genomic insights. Genomics. 2021;113(6):3951-3966. CrossRef PubMed
- 106. Howard R, Zubko O, Bradley R, et al; Minocycline in Alzheimer Disease Efficacy (MADE) Trialist Group. Minocycline at 2 different dosages vs placebo for patients with mild Alzheimer disease: a randomized clinical trial. JAMA Neurol. 2020;77(2):164-174. CrossRef PubMed
- 107. Gandra S, Mojica N, Klein EY, et al. Trends in antibiotic resistance among major bacterial pathogens isolated from blood cultures tested at a large private laboratory network in India, 2008-2014. Int J Infect Dis. 2016;50(50):75-82. CrossRef PubMed
- 108. Narendrakumar L, Chandrika SK, Thomas S. Adaptive laboratory evolution of Vibrio cholerae to doxycycline associated with spontaneous mutation. Int J Antimicrob Agents. 2020;56(3):106097. CrossRef PubMed
- 109. Kotwani A, Joshi J, Lamkang AS. Over-the-counter sale of antibiotics in India: a qualitative study of providers’ perspectives across two states. Antibiotics (Basel). 2021;10(9):1123. CrossRef PubMed
- 110. Fair RJ, Tor Y. Antibiotics and Bacterial Resistance in the 21st century. Perspect. Medicin Chem; 2014;6:25-64.
- 111. Yacouba A, Olowo-Okere A, Yunusa I. Repurposing of antibiotics for clinical management of COVID-19: a narrative review. Ann Clin Microbiol Antimicrob. 2021;20(1):37-38. CrossRef PubMed
- 112. Burns AL, Sleebs BE, Gancheva M, et al. Targeting malaria parasites with novel derivatives of azithromycin. Front Cell Infect Microbiol. 2022;12:1063407. CrossRef PubMed
- 113. Burns AL, Sleebs BE, Siddiqui G, et al. Retargeting azithromycin analogues to have dual-modality antimalarial activity. BMC Biol.2020;18:1-23.
- 114. Gore-Langton GR, Cairns M, Compaoré YD, et al. Effect of adding azithromycin to the antimalarials used for seasonal malaria chemoprevention on the nutritional status of African children. Trop Med Int Health. 2020;25(6):740-750.
- 115. Peric M, Pešić D, Alihodžić S, et al. A novel class of fast-acting antimalarial agents: substituted 15-membered azalides. Br J Pharmacol. 2021;178(2):363-377. CrossRef PubMed
- 116. Advani D, Kumar P. Therapeutic targeting of repurposed anticancer drugs in Alzheimer’s disease: using the multiomics approach. ACS Omega. 2021;6(21):13870-13887. CrossRef PubMed
- 117. Tímár J, Ladányi A, Forster-Horváth C, et al. Neoadjuvant immunotherapy of oral squamous cell carcinoma modulates intratumoral CD4/CD8 ratio and tumor microenvironment: a multicenter phase II clinical trial. J Clin Oncol. 2005;23(15):3421-3432. CrossRef PubMed
- 118. Avershina E, Shapovalova V, Shipulin G. Fighting antibiotic resistance in hospital-acquired infections: current state and emerging technologies in disease prevention, diagnostics and therapy. Front Microbiol. 2021;12:2044.
- 119. Tyers M, Wright GD. Drug combinations: a strategy to extend the life of antibiotics in the 21st century. Nat Rev Microbiol. 2019;17(3):141-155. CrossRef PubMed
- 120. Hartkoorn RC, Sala C, Neres J, et al. Towards a new tuberculosis drug: pyridomycin-nature’s isoniazid. EMBO Mol Med. 2012;4(10):1032-1042.
- 121. Diacon AH, Dawson R, Groote-Bidlingmaier F, et al. Bactericidal activity of pyrazinamide and clofazimine alone and in combinations with pretomanid and bedaquiline. Am J Respir Crit Care Med. 2015;191(8):943-953.
- 122. Weng H-B, Chen H-X, Wang M-W. Innovation in neglected tropical disease drug discovery and development. Infect Dis Poverty. 2018;7(1):67. CrossRef PubMed
- 123. Lauring AS, Andino R. Quasispecies theory and the behavior of RNA viruses. PLoS Pathog. 2010;6(7):e1001005. CrossRef PubMed
- 124. Ventola CL. The antibiotic resistance crisis: part 1: causes and threats. P&T. 2015;40(4):277-283. PubMed
- 125. Scherf A, Lopez-Rubio JJ, Riviere L. Antigenic variation in Plasmodium falciparum. Annu Rev Microbiol. 2008;62:445-470.
- 126. Gomez JE, McKinney JD. M. tuberculosis persistence, latency, and drug tolerance. Tuberculosis (Edinb). 2004;84(1-2):29-44. CrossRef PubMed
- 127. Barrett MP, Kyle DE, Sibley LD, Radke JB, Tarleton RL. Protozoan persister-like cells and drug treatment failure. Nat Rev Microbiol. 2019;17(10):607-620. CrossRef PubMed
- 128. Donlan RM. Biofilms and device-associated infections. Emerg Infect Dis. 2001;7(2):277-281. CrossRef PubMed
- 129. Hay M, Thomas DW, Craighead JL, Economides C, Rosenthal J. Clinical development success rates for investigational drugs. Nat Biotechnol. 2014;32(1):40-51. CrossRef PubMed
- 130. Ginsberg AM, Spigelman M. Challenges in tuberculosis drug research and development. Nat Med. 2007;13(3):290-294. CrossRef PubMed
- 131. Takuadina AI, Pazylkhan NP, Iskakov KT. Mathematical modeling of infectious diseases on the example of Astana city. Sci Educ. 2023;71.
- 132. Jiménez-Díaz MB, Mulet T, Viera S, et al. Improved murine model of malaria using Plasmodium falciparum competent strains and non-myelodepleted NOD-SCID IL2Rgammanull mice engrafted with human erythrocytes. Antimicrob Agents Chemother. 2009;53(10):4533-4536. CrossRef PubMed
- 133. Aguilar C, Alves da Silva M, Saraiva M, et al. Organoids as host models for infection biology – a review of methods. Exp Mol Med. 2021;53(10):1471-1482. PubMed
- 134. Mihaljevic JR, Borkovec S, Ratnavale S, et al. SPARSEMODr: rapidly simulate spatially explicit and stochastic models of COVID-19 and other infectious diseases. Biol Methods Protocols. 2022;7(1):bpac022.
- 135. Blutt SE, Estes MK. Organoid models for infectious disease. Annu Rev Med. 2022;73(1):167-182. CrossRef PubMed
- 136. Smith GC, Kao RR, Walker M. Infectious disease modelling to inform policy. Rev Sci Tech. 2023;42:173-179. CrossRef PubMed
- 137. Anna PGS, Henrique dos Santos M, Banerjee A. A statistical examination of distinct characteristics influencing the performance of vector-borne epidemiological agent-based simulation models. Modelling. 2021;2(2):166-196. CrossRef
- 138. Raja D. The power of epidemiological modelling in understanding and managing infectious diseases. Chettinad Health City Med J (E-2278-2044 & P-2277-8845), 2023;12(1):1-2.
- 139. Rong N, Liu J. Development of animal models for emerging infectious diseases by breaking the barrier of species susceptibility to human pathogens. Emerg Microbes Infections. 2023;12(1):2178242.
- 140. Fitzpatrick MC, Bauch CT, Townsend JP, Galvani AP. Modelling microbial infection to address global health challenges. Nat Microbiol. 2019;4(10):1612-1619. CrossRef PubMed
- 141. Cartelle Gestal M, Dedloff MR, Torres-Sangiao E. Computational health engineering applied to model infectious diseases and antimicrobial resistance spread. Appl Sci (Basel). 2019;9(12):2486. CrossRef
- 142. Legrand N, Ploss A, Balling R, et al. Humanized mice for modeling human infectious disease: challenges, progress, and outlook. Cell Host Microbe 2009;6(1):5-9.
- 143. Swearengen JR. Choosing the right animal model for infectious disease research. Animal Model Exp Med. 2018;1(2):100-108. CrossRef PubMed