![]() |
Drug Target Insights 2023; 17: 5-30 ISSN 1177-3928 | DOI: 10.33393/dti.2023.2477 REVIEW |
![]() |
A systematic review of mucoadhesive vaginal tablet testing
ABSTRACT
Drug administration through the vaginal tract is one of the oldest modalities of pharmacotherapy, and it is also one of the most explored. Since the vaginal cavity has a wide surface area, a plentiful blood supply, and a complex network of blood arteries, it can evade hepatic first-pass metabolism and obtain high local drug concentrations. Vaginal pills look to be a good dose form since they are simple to use, portable, and can easily deliver the required amount of medicine. Vaginal formulations, on the other hand, are vulnerable to rapid expulsion due to the vaginal tract’s self-cleaning action, which reduces the formulation’s efficiency. Currently, there is an increasing amount of focus on mucoadhesive vaginal formulation research and development to fix the formulation at the place where the medicine can be released and/or absorbed. This article examines all of the strategies used by researchers to develop a mucoadhesive vaginal tablet that is safe, effective, and comfortable for the user.
Keywords: Dissolution, Mucoadhesion, Physicochemical, Swelling
Received: July 27, 2022
Accepted: December 7, 2022
Published online: January 16, 2023
Drug Target Insights - ISSN 1177-3928 - www.aboutscience.eu/dti
© 2023 The Authors. This article is published by AboutScience and licensed under Creative Commons Attribution-NonCommercial 4.0 International (CC BY-NC 4.0).
Commercial use is not permitted and is subject to Publisher’s permissions. Full information is available at www.aboutscience.eu
Introduction
Over the past three decades, the vaginal route has gained relevance in modern medicine as a route for drug delivery and is now considered an option for several therapeutic strategies, specifically for female-related conditions. Several advantages have been claimed for vaginal drug delivery in managing local conditions and achieving systemic effects. In the case of managing local conditions, vaginal administration means that lower doses can be effective (compared to the oral route), which frequently leads to reduced systemic exposure and can prevent side effects (1,2). Owing to its large surface area, rich blood supply, and the presence of a dense network of blood vessels, the vagina serves as a promising site for systemic drug delivery (3). Its relatively high permeability to many drug compounds (including several with high molecular weight) also allows for drug transport across the vaginal mucosa and access into the blood circulation, presenting in many cases higher flux levels than those observed via intestinal tissues (2,4). The vaginal route may prove to be of particular importance in the case of drugs undergoing extensive hepatic metabolism, since it avoids the hepatic first-pass effect (1). Furthermore, it permits the elimination of possible degradation in the gastrointestinal tract and the effect of the drug directly at the site of application (5).
Traditionally, the vaginal route has been used for delivery of locally acting drugs such as antibacterial, antifungal, antiprotozoal, antiviral, labor-inducing, and spermicidal agents, prostaglandins, and steroids (6). Recently, there has been increased interest and effort in the development of vaginal formulations such as microbicides that provide effective contraception and protection against transmission of various sexually transmitted diseases (STDs), including acquired immunodeficiency syndrome (AIDS) (3). There are many different vaginal products in the market to treat different vaginal conditions, for example, Canesten® for antifungal and Nuvaring® as contraceptive (7,8). One of the leading vaginal tablets available in the market is Vagifem® by Novo Nordisk, which was first introduced in 1988. It is an option for local estrogen therapy that continuously releases steroid in the vagina for a consistent dose of hormone (9). There are many other vaginal tablets that are currently in the process of patent application and are listed in Table I. These applications are in various stages of the patenting process, some has been granted and anticipating expiry, some are still pending, and some have just published a research article under the patent ID.
Patent ID | Author/inventor | Title | Treatment/condition | Date of application | Current status |
---|---|---|---|---|---|
CN1307986C | Chen Z. | Vaginal effervescent tablets for inflammation and their preparation | Gynecological inflammation | 2004-06-08 | Granted (2007-04-04) Anticipated expiration (2024-06-08) |
AU2012210296B2 | Mogna G., Mogna L., Strozzi G.P. | Effervescent composition in solid form for use in vaginal application for the treatment of vaginal infections | Vaginal infections | 2011-01-28 | Granted (2017-01-05)
Anticipated expiration (2032-01-24) |
CN106420726A | Jiang Dingyu, Zhang Yongwei, Zhu Ming | Clotrimazole vaginal tablets | Vulvovaginal candidiasis | 2016-06-30 | Pending |
WO2020201515A1 | Ellervik U., Manner S., Sterner O., Strevens H., Lindberg N., Säfholm A. | Vaginal tablet formulation | Vaginal microbial infections | 2019-04-05 | Publication (2020-10-08) |
US20200155621A1 | Thoral C., Tchoreloff P., Mazel V., Busignies V., Nivoliez A. | Mucoadhesive sustained-release vaginal tablet | Probiotic strain: Lactobacillus | 2014-03-10 | Pending |
W02022218487A1 | Crouzier T., Schimpf U. | A vaginal contraceptive composition for reinforcement of the cervical mucus barrier properties | Contraceptive | 2021-04-12 | Publication (2022-10-20) |
These patents have been sourced from Google Patent and accessed on November 28, 2022.
Vaginal products do not need to be sterile; they are usually cheap and relatively easy to manufacture (2). It also allows easy and comfortable self-administration and rarely requires the intervention of a health-care provider (1). Marketed vaginal dosage forms include solutions (douches), semisolids (creams, ointments, and gels), and solid formulations (tampons, capsules, pessaries, suppositories, films, sponges, powders, and special controlled release devices like the intravaginal ring) as well as other types of formulations such as aerosols and particulate systems integrated in adequate drug delivery systems (3,6,10). However, the use of vaginal formulations can be limited due to poor drug retention in the vaginal tract, as they are removed in a short time by the tract’s self-cleansing action (3). The low residence time often leads to disappointing experiences such as leakages and messiness, which cause loss of formulation from the application site, giving rise to inadequate formulation and hence lack of effectiveness (11). Therefore, frequent daily doses are often required to maintain an effective drug concentration, which further complicates application and contributes to low patient acceptability and, thus, poor compliance (3,4,6,12,13).
Extensive research and innovative attempts have been made to develop vaginal formulations to meet clinical and user requirements. To overcome these limitations, researchers have focused their attention on the development of new delivery systems that can prolong the drug residence time in the vaginal cavity, basically by using mucoadhesive formulations (3,12,14,15). The general principles of the mucoadhesive vaginal drug delivery system will be discussed further in a later section of this review.
Mucoadhesive vaginal drug delivery systems are superior to conventional ones due to their ability to prolong drug residence time at the application site, leading to improved bioavailability and efficacy (12). The number of products based on new vaginal drug delivery systems has significantly increased, and this growth is expected to continue in the near future (3). With various types of formulations available, the popularity of vaginal products can be different among women from different backgrounds and countries (6). Nonetheless, tablets and gels (films) are among the most popular vaginal formulations (3,6,12). Even with major advancement in the gel (hydrogel) and film formulations, moderate vaginal leakage was still observed and daily administration was required (16). Therefore, vaginal tablets still often represent the typically acceptable dosage form with stability-related advantages and an economical choice for both manufacturers and users (17,18), thus advocating its role and relevance in the vaginal drug delivery system. So, it won’t be obsolete just yet.
This review aims to assemble and discuss the key parameters and unique methodologies that should be considered when evaluating vaginal tablet formulations. This review will not go into any specific medicinal substances or polymers; instead, it will concentrate on the tactics and evaluation methods that can aid in the development of an effective vaginal tablet formulation. To the author’s knowledge, this is the first systematic review that compiles numerous assessment methodologies required to develop a new mucoadhesive vaginal tablet formulation.
Methods
Eligibility criteria
Inclusion criteria
i. All original studies designing and formulating mucoadhesive vaginal tablets, regardless of its therapeutic treatment
ii. Publication years between 2000 and November 2021
iii. Articles in English language
iv. Articles published in scholarly peer-reviewed journals
Exclusion criteria
i. Articles of studies on other mucoadhesive vaginal dosage forms (e.g., semisolids and liquids)
ii. Articles of studies on vaginal pessaries, suppositories, and pellets because it has been identified that they are different from tablet dosage forms
iii. Articles of studies of mucoadhesive vaginal tablet for veterinary use
iv. Articles of studies that compare between two different dosage forms (e.g., tablets and films)
Information sources
A search of relevant papers between years 2000 and 2021 was made via chosen electronic databases available in the Technology University of Shannon: Midlands Midwest (TUS) Library online search engine. The databases included PubMed, Science Direct, Multidisciplinary Digital Publishing Institute (MDPI) and Academic Search Complete (Ebsco). The search was done between August 19 and November 11, 2021, for any new relevant publications.
Search strategy
The search in the databases was carried out using the keyword “mucoadhesive vaginal tablets” or “vaginal tablets + mucoadhesive” or “vaginal tablets + evaluations” or “vaginal tablet testing + mucoadhesion.”
Study selection and data collection process
The initial stage involves screening the titles. Titles that specify a dosage form other than vaginal tablets (e.g., films, gels) and for veterinary purposes are essentially excluded. In the case of vague titles, a quick scan through the abstract is conducted to identify the words, vaginal tablet or tablet/s. Titles that do not use the term “vaginal tablet” are considered as vague titles. Following that, the abstracts were screened to ensure that they meet the inclusion criteria. The full text of eligible abstracts is then accessed and reviewed.
Two reviewers were involved in the selection process. Articles were collected individually; the other reviewer replicated the search strategy and listed the articles deemed eligible for the review. Excluded articles from each reviewer were confirmed with each other and eligible articles are compiled. Studies that were deemed eligible were compiled in an Excel spreadsheet (Microsoft 365 application, version 2201). Data were collected systematically and analyzed using Excel spreadsheet.
Data items
Data extracted from each study include: (i) information of the tablets (e.g., use, physical appearance, etc.), (ii) all the methods used to measure the physicochemical properties of the tablets, (iii) all the methods used to assess the mucoadhesion property of the tablet, (iv) all the methods used to evaluate drug release profile of the tablet, and (v) other relevant methods used to evaluate the technical working ability of the tablet. For each outcome, effect measures were determined via difference in means and standard deviation. All graphs to presenting the results were prepared using Excel software (Microsoft 365 application, version 2201).
Study risk of bias assessment
Each article was assessed for risk of bias using the Cochrane Collaborations tool (19). The following biases were examined: (i) bias in selection/sampling, (ii) performance bias associated with the allocation of interventions during the study, (iii) attrition bias associated with the handling of incomplete outcome data, (iv) reporting bias associated with selective outcome reporting, (v) measurement bias associated with the use of non-validated data collection criteria, and (vi) analysis bias associated with the omission of necessary statistical coefficients associated with the study.
Synthesis methods
The full text of each article was scrutinized in sequence and grouped according to the data items mentioned in Section 2.5. Data were collected systematically and tabulated and analyzed (means and standard deviation) using Excel software (Microsoft 365 application, version 2201). All graphs prepared to present the results were prepared using the same software.
Results
Study selection
There were 772 research articles that were identified as a result of the keyword search of mucoadhesive vaginal tablets across five electronic databases. The breakdown is as follows: (i) PubMed, 65 articles; (ii) Science Direct, 624 articles; (iii) MDPI, 5 articles; (iv) Scopus, 77 articles, and (v) Ebsco, 1 article. From the initial screening of the titles, a total of 63 research articles were deemed eligible to proceed for abstract screening. At this stage, the research articles were sub-divided into two categories according to the study characteristic: (i) research articles with a single formulation of vaginal tablet (study of inter-batches variation) and (ii) vaginal tablets formulation with polymer intervention to improving the mucoadhesive properties of the formulation (comparison study between modified and non-modified formulations). The subcategories were to facilitate the reviewers in understanding the study design and characteristic of the articles. Following the abstract screening, 43 research articles were deemed eligible to proceed for full-text review and data collection. For most excluded research articles, the study focuses more on a specify drug compound or polymer rather than the technology of tablet manufacturing and performance. In addition, four other research articles were then excluded during the full-text review, due to one being outdated (20) and three research articles having non-vaginal tablet-related outcomes (21-23). In these research articles, most formulations were simply made into KBr disks to test the mucoadhesive property exclusively, no further tablet manufacturing parameters were considered. Thus, it is considered non-vaginal tablet-related outcomes. To avoid further limitations, unaccessible research articles were agreed to not be included in this review. Figure 1 shows the flow diagram of the selection process and that at the end of this process, 39 research articles were included in this review.
Study characteristics
Table II summarizes the study characteristics of the research articles reviewed. All of the vaginal tablets were prepared by direct compression, with most having flat-faced and round or cylindrical shape. More of the study characteristics are discussed in Section 4.2.
The studies were divided into two subcategories depending on the study design: either (a) inter-batch comparison or (b) polymer intervention. The study background of the articles reviewed is summarized in Table III. Inter-batch comparison studies involved vaginal tablet formulations that were prepared in different batches varying in different types and/or ratios, and/or combinations of mucoadhesive polymers in each batch. Some are natural polymers, some in combination with synthetics ones. In addition, there were some articles that compared different batches that were made using solutions with different pH (13), some with different modification of the drug compound itself instead of the polymer (24). On the other hand, studies with polymer intervention involved modification to a selected mucoadhesive polymer into thiomers. Thiomers (thiolated polymers) are currently thought to be a new generation of mucoadhesive polymers, as they have thiol group side chains that can form inter- and/or intrachain disulfide bonds and improve the cohesive property of a formulation (25). Therefore, these studies include tablet evaluations comparing between batches formulated using non-thiolated and thiolated polymers.
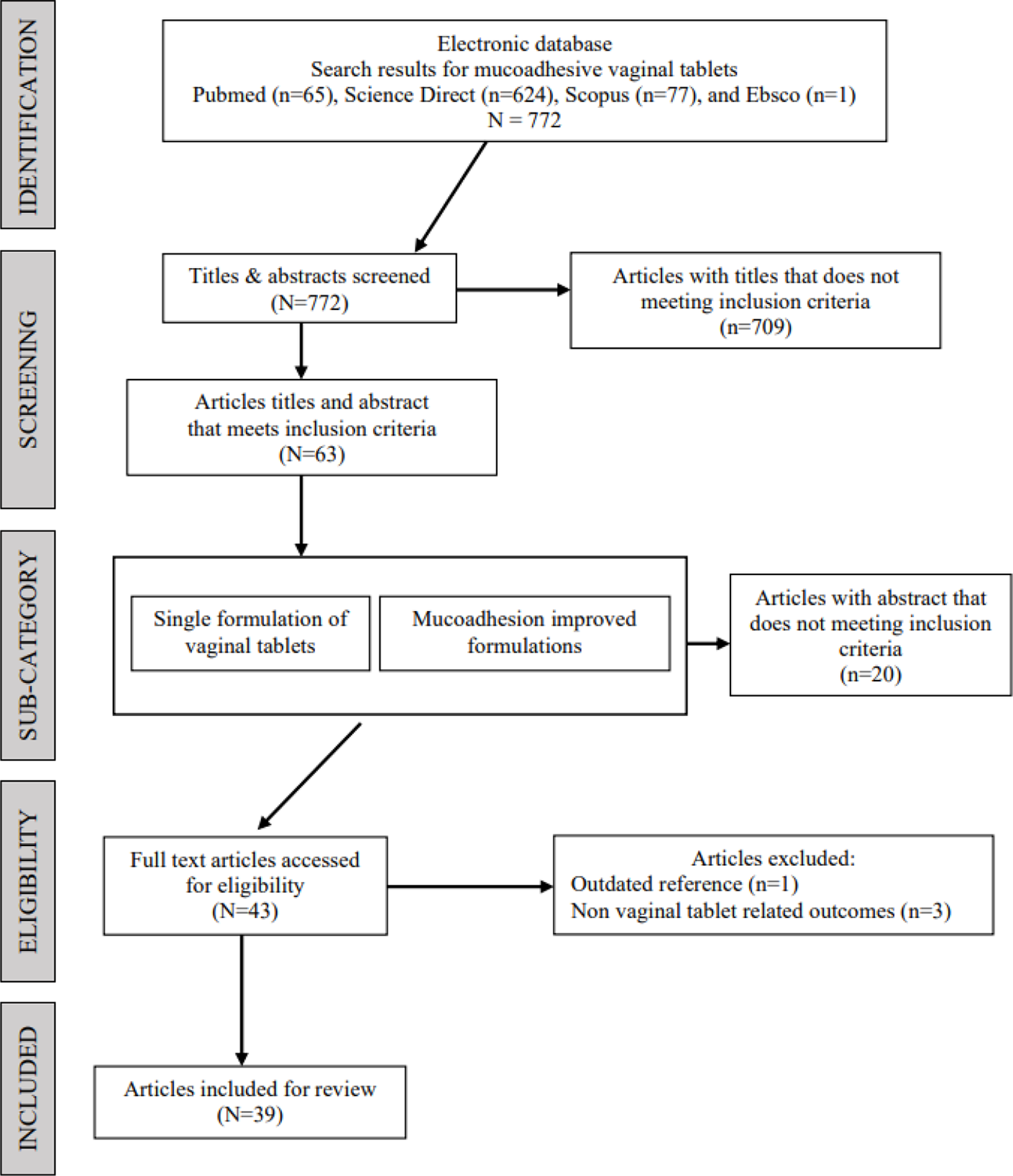
Fig. 1 - PRISMA flow diagram of the study selection process.
Research article | Author | Study design | Vaginal tablet design | Treatment application | Study title |
---|---|---|---|---|---|
1 | Abidin et al (2020) | Inter-batch comparison (n* = 6) | Bilayer tablet, flat faced, round shaped | Anticancer | A bilayer vaginal tablet for the localized delivery of disulfiram and 5-fluorouracil to the cervix |
2 | Abu El-Enin et al (2020) | Inter-batch comparison (n* = 6) | Core-in-cup tablet flat faced, round shaped | Preterm labor | Formulation, development, in vivo pharmacokinetics and pharmacological efficacy evaluation of novel vaginal bioadhesive sustained core-in-cup salbutamol sulphate tablets for preterm labor |
3 | Baki et al (2009) | Inter-batch comparison (n* = 6) | Flat faced, round shaped | Vaginal health management | Formulation of a solid intravaginal matrix system to prolong the pH-decreasing effect of lactic acid |
4 | Baloglu et al (2011) | Polymer intervention | Flat faced, round shaped | Antifungal | In vitro evaluation of mucoadhesive vaginal tablets of antifungal drugs prepared with thiolated polymer and development of a new dissolution technique for vaginal formulations |
5 | Bartkowiak et al (2018) | Inter-batch comparison (n* = 6) | Flat faced, round shaped | Antifungal | Surface and swelling properties of mucoadhesive blends and their ability to release fluconazole in a mucin environment |
6 | Bhat et al (2010) | Inter-batch comparison (n* = 6) | Flat faced, round shaped | Vaginal infection | Bioadhesive controlled release clotrimazole vaginal tablets |
7 | Cazorla-Luna et al (2019) | Inter-batch comparison (n* = 15) | Flat faced, round shaped | HIV prevention | Chitosan-based mucoadhesive vaginal tablets for controlled release of the anti-HIV drug tenofovir |
8 | Cevher et al (2014) | Inter-batch comparison (n* = 4) | Flat faced, round shaped | Antifungal | Bioadhesive tablets containing cyclodextrin complex of itraconazole for the treatment of vaginal candidiasis |
9 | Cevher et al (2008) | Inter-batch comparison (n* = 3) | Flat faced, round shaped | Antifungal | Preparation and characterisation of natamycin: g-cyclodextrin inclusion complex and its evaluation in vaginal mucoadhesive formulations |
10 | El-Kamel et al (2002) | Inter-batch comparison (n* = 4) | Flat faced, round shaped | Antibacterial | Chitosan and sodium alginate–based bioadhesive vaginal tablets |
11 | Fitaihi et al (2017) | Inter-batch comparison (n* = 17) | Flat faced, round shaped | Antifungal | Role of chitosan on controlling the characteristics and antifungal activity of bioadhesive fluconazole vaginal tablets |
12 | Gupta et al (2013) | Inter-batch comparison (n* = 6) | Flat faced, round shaped | Antimycotic | Bioadhesive vaginal tablets containing spray dried microspheres loaded with clotrimazole for treatment of vaginal candidiasis |
13 | Gök et al (2017) | Polymer intervention | Flat faced, round shaped | Did not specify | The effects of the thiolation with thioglycolic acid and l-cysteine on the mucoadhesion properties of the starch-graft-poly(acrylic acid) |
14 | Hani et al (2016) | Inter-batch comparison (n* = 10) | Flat faced, round shaped | Antifungal | Development of a curcumin bioadhesive monolithic tablet for treatment of vaginal candidiasis |
15 | Hassan et al (2017) | Inter-batch comparison (n* = 12) | Flat faced, round shaped | Hormone therapy | Mucoadhesive tablets for the vaginal delivery of progesterone: in vitro evaluation and pharmacokinetics/pharmacodynamics in female rabbits |
16 | Hombach et al (2009) | Polymer intervention | Flat faced, round shaped | Vaginal infection | Development and in vitro evaluation of a mucoadhesive vaginal delivery system for nystatin |
17 | Kailasam et al (2010) | Inter-batch comparison (n* = 5) | Flat faced, round shaped | Antibacterial | Formulation and evaluation of once daily mucoadhesive vaginal tablet of metronidazole |
18 | Kast et al (2002) | Polymer intervention | Flat faced, round shaped | Vaginal infection | Design and in vitro evaluation of a novel bioadhesive vaginal drug delivery system for clotrimazole |
19 | Khan et al (2017) | Inter-batch comparison (n* = 8) | Did not specify | HIV prevention | Formulation and evaluation of once daily mucoahdesive vaginal tablet of metronidazole |
20 | Khan et al (2014) | Inter-batch comparison (n* = 8) | Did not specify | HIV prevention | Formulation and evaluation of mucoadhesive vaginal tablets of tenofovir disoproxil fumarate |
21 | Lupo et al (2017) | Polymer intervention | Flat faced, round shaped | Antibacterial | Entirely S-protected chitosan: a promising mucoadhesive excipient for metronidazole vaginal tablets |
22 | Notario-Pérez et al (2019) | Inter-batch comparison (n* = 5) | Flat faced, round shaped | HIV prevention | Tenofovir hot-melt granulation using Gelucire® to develop sustained-release vaginal systems for weekly protection against sexual transmission of HIV |
23 | aNotario-Pérez et al (2017) | Inter-batch comparison (n* = 12) | Flat faced, round shaped | HIV prevention | Optimization of Tenofovir release from mucoadhesive vaginal tablets by polymer combination to prevent sexual transmission of HIV |
24 | bNotario-Pérez et al (2017) | Inter-batch comparison (n* = 12) | Flat faced, round shaped | HIV prevention | Influence of chitosan swelling behaviour on controlled release of tenofovir from mucoadhesive vaginal systems for prevention of sexual transmission of HIV |
25 | Nowak et al (2015) | Polymer intervention | Flat faced, round shaped | Did not specify | Preactivated hyaluronic acid: a potential mucoadhesive polymer for vaginal delivery |
26 | Pacheco-Quito et al (2020) | Inter-batch comparison (n* = 8) | Flat faced, round shaped | Vaginal infection | Carrageenan-based acyclovir mucoadhesive vaginal tablets for prevention of genital herpes |
27 | Paczkowska et al (2020) | Inter-batch comparison (n* = 6) | Did not specify | Vaginal infection | Mucoadhesive chitosan delivery system with Chelidonii herba lyophilized extract as a promising strategy for vaginitis treatment |
28 | Palade et al (2013) | Inter-batch comparison (n* = 12) | Did not specify | Did not specify | In vitro evaluation of 5-fluorouracil dissolution profiles from vaginal bioadhesive tablets |
29 | Patel A. et al (2012) | Inter-batch comparison (n* = 9) | Did not specify | Antifungal | Design, development and in vitro evaluation of sertaconazole mucoadhesive vaginal tablet |
30 | Patel A. et al (2011) | Inter-batch comparison (n* = 10) | Did not specify | Antifungal | Development and evaluation of mucoadhesive vaginal tablet of sertaconazole for vaginal candidiasis |
31 | Patel G.M. et al (2010) | Inter-batch comparison (n* = 9) | Did not specify | Antifungal | A novel effervescent bioadhesive vaginal tablet of ketoconazole: formulation and invitro evaluation |
32 | Pendekal et al (2013) | Inter-batch comparison (n* = 9) | Flat faced, round shaped | Anticancer | Hybrid drug delivery system for oropharyngeal, cervical and colorectal cancer—in vitro and in vivo evaluation |
33 | Pendekal et al (2012) | Inter-batch comparison (n* = 9) | Flat faced, round shaped | Anticancer | Development and characterization of chitosan-polycarbophil interpolyelectrolyte complex-based 5-fluorouracil formulations for buccal, vaginal and rectal application |
34 | Perioli et al (2009) | Inter-batch comparison (n* = 6) | Flat faced, round shaped | Antibacterial | FG90 chitosan as a new polymer for metronidazole mucoadhesive tablets for vaginal administration |
35 | Perioli et al (2011) | Inter-batch comparison (n* = 5) | Flat faced, round shaped | Vaginal infection | New solid mucoadhesive systems for benzydamine vaginal administration |
36 | Sánchez et al (2017) | Inter-batch comparison (n* = 4) | Double layer, flat faced, round shaped | Vaginal infection | A novel double-layer mucoadhesive tablet containing probiotic strain for vaginal administration: design, development and technological evaluation |
37 | Szymańska et al (2014) | Inter-batch comparison (n* = 4) | Flat faced, round shaped | Vaginal infection | Vaginal chitosan tablets with clotrimazole—design and evaluation of mucoadhesive properties using porcine vaginal mucosa, mucin and gelatine |
38 | Tunpanich et al (2019) | Inter-batch comparison (n* = 5) | Capsule shaped | Hormone therapy | Mucoadhesive sustained-release tablets for vaginal delivery of Curcuma comosa extracts: preparation and characterization |
39 | Valenta et al (2001) | Polymer intervention | Flat faced, round shaped | Hormone therapy | Development and in vitro evaluation of a mucoadhesive vaginal delivery system for progesterone |
HIV = human immunodeficiency virus.
*n = number of batches formulated and compared to.
Author | Study background | Polymers used |
---|---|---|
Abidin et al (2020) | Designed a bilayer vaginal tablet. A comparison study between batches formulated using different ratios of polymers in individual layers. | Chitosan, poly(acrylic acid) |
Abu El-Enin et al (2020) | Designed a core-in-cup vaginal tablet. A comparison study between batches formulated using different combinations of mucoadhesive polymers. | Carbopol, hydroxypropyl methylcellulose, hydroxyethylcellulose, polyethylene glycol |
Baki et al (2009) | Studied the applicability of solid matrix system for tablet formulations. A comparison study between batches formulated with different concentrations of API. | Hydroxypropyl methylcellulose, microcrystalline cellulose |
Baloglu et al (2011) | A comparison study between batches formulated using thiolated and non-thiolated mucoadhesive polymers. Article includes polymer modification steps and structure characterization methods. | Poly(acrylic acid), poly(acrylic acid)-cysteine (thiolated) |
Bartkowiak et al (2018) | A comparison study between batches formulated using different blends of mucoadhesive polymers. | Carbopol, polycarbophil, chitosan, hydroxyethylcellulose, hydroxypropyl methylcellulose |
Bhat et al (2010) | A comparison study between batches formulated using mixtures of natural mucoadhesive polymer with HPMC in different ratios. | Hydroxypropyl methylcellulose, sodium carboxymethylcellulose, guar gum |
Cazorla-Luna et al (2019) | A comparison study between batches formulated using CHN alone and in combination with natural mucoadhesive polymers. The different blends of polymers were used to generate spontaneous polyelectrolyte complexes. Article includes structure characterization methods of the complexes formed. | Chitosan, pectin, locust bean gum |
Cevher et al (2014) | This study focuses on forming inclusion complex of cyclodextrin with the chosen API to improve their aqueous solubility. The complex was then formulated into sustained-release vaginal tablet. | Hydroxypropyl methylcellulose, carbopol, xanthan gum |
Cevher et al (2008) | A comparison study between batches formulated using different polymer ratios. This study focuses on forming inclusion complex of cyclodextrin with the chosen API to improve their aqueous solubility. The complex was then formulated with a polymer at different ratios until high mucoadhesion to the vaginal mucosa is achieved. | Hydroxypropyl methylcellulose, carbopol, xanthan gum |
El-Kamel et al (2002) | A comparison study between batches formulated using mixtures of anionic polymers with CHN. The polymer combinations were used to generate spontaneous polyelectrolyte complexes. | Chitosan, microcrystalline cellulose, sodium carboxymethylcellulose, sodium alginate |
Fitaihi et al (2017) | A comparison study between batches formulated using different types of polymers physically blended with CHN at different ratios. | Chitosan, hydroxypropyl methylcellulose, guar gum, sodium carboxymethylcellulose, polyvinyl pyrrolidone |
Gupta et al (2013) | Incorporated microspheres of the API into a vaginal tablet formulation. A comparison study between batches formulated using the API in the pure form and the API microspheres. | Eudragit RS®, Eudragit RL, carbopol, hydroxypropyl methylcellulose, sodium carboxymethylcellulose |
Gök et al (2017) | This study investigated the effect of thiolation reagents on the thiolation process. Article includes polymer modification and confirmation methods. A comparison study between batches formulated using thiolated and non-thiolated mucoadhesive polymers. | Poly(acrylic acid), poly(acrylic acid)-cysteine (thiolated), poly(acrylic acid)-thioglycolic acid (thiolated) |
Hani et al (2016) | A comparison study between batches formulated using different types of polymers and at different ratios. | Hydroxypropyl methylcellulose, xantham gum, guar gum |
Hassan et al (2017) | A comparison study between batches formulated using different types of polymers. | Carbopol, hydroxypropyl methylcellulose, chitosan, sodium alginate |
Hombach et al (2009) | A comparison study between batches formulated using two different thiolated polymers (thiomers); the conjugates of poly(acrylic acid). | Poly(acrylic acid), poly(acrylic acid)-cysteine, poly(acrylic acid)-cysteamine |
Kailasam et al (2010) | A comparison study between batches formulated using wo different types of polymer in different ratios. | Carbopol, hydroxypropyl methylcellulose |
Kast et al (2002) | Investigated the effect of thiolation by using various amounts of thiolating agent. A comparison study between batches formulated using thiolated and non-thiolated polymers. | Chitosan, chitosan-thioglycolic acid (thiolated) |
Khan et al (2017) | Investigated the application of PCP as the matrix forming polymer with other types of polymers. A comparison study between batches formulated with different polymer combinations. | Carbopol, chitosan, sodium carboxymethylcellulose |
Khan et al (2014) | Formulation and evaluation of mucoadhesive vaginal tablets of tenofovir disoproxil fumarate. | Hydroxypropyl methylcellulose, carbopol, chitosan, sodium carboxymethylcellulose |
Lupo et al (2017) | Investigated the effect of enhanced modification by protecting the thiol groups on thiolated polymers to avoid oxidation and increase the stability of the polymer. A comparison study between batches formulated using S-protected thiomer and non-thiolated polymer. | Chitosan, S-protected chitosan |
Notario-Pérez et al (2019) | Evaluated and compared batches of vaginal tablets formulated using granules prepared by hot-melt granulation method. | Chitosan, hydroxypropyl methylcellulose, polyvinyl pyrrolidone |
Notario-Pérez et al (a) (2017) | A comparison study between batches formulated using different polymer combinations. | Hydroxypropyl methylcellulose, chitosan, Eudragit RS®, guar gum |
Notario-Pérez et al (b) (2017) | A comparison study between batches formulated using natural, semisynthetic, and synthetic polymers. | Chitosan, hydroxypropyl methylcellulose, Eudragit RS®, guar gum |
Nowak et al (2015) | Investigated the effect of enhanced modification by using preactivated thiomer on polymer stability. | Hyaluronic acid-cysteine-6-mercaptonicotinamide |
Pacheco-Quito et al (2020) | A comparison study between batches formulated using different types of polymers and at different amount. | Iota-carrageenan, hydroxypropyl methylcellulose |
Paczkowska et al (2020) | Developed vaginal drug delivery system using lyophilized extract with CHN carrier. | Chitosan, hydroxypropyl methylcellulose, microcrystalline cellulose |
Palade et al (2013) | A comparison study between batches formulated using acrylic acid derivatives and cellulose derivatives. | Carbopol, metolose |
Patel A. et al (2012) | A comparison study between batches formulated with different combination of polymers. In addition, this study included an effervescent feature in the formulation to enhance the swelling of the tablets. | Carbopol, chitosan, sodium carboxymethylcellulose, methyl cellulose, hydroxypropyl methylcellulose, hydroxyethylcellulose, sodium alginate |
Patel A. et al (2011) | A comparison study between batches formulated with different combination of polymers. In addition, this study included an effervescent feature in the formulation to enhance the swelling of the tablets. | Carbopol, chitosan, sodium carboxymethylcellulose, methyl cellulose, hydroxypropyl methylcellulose, hydroxyethylcellulose, sodium alginate |
Patel G.M. et al (2010) | A comparison study between batches formulated with different combination of polymers. In addition, this study included an effervescent feature in the formulation to enhance the swelling of the tablets. | Hydroxypropyl methylcellulose, carbopol, sodium carboxymethylcellulose, chitosan, methyl cellulose, sodium alginate |
Pendekal et al (2013) | This study investigated spontaneous interpolymer complexes between CHN and alginate. It is thought to be a newer and efficient form of polymeric carriers and will make the tablet more versatile in its application. | Chitosan, alginate |
Pendekal et al (2012) | This study investigated spontaneous interpolymer complexes between CHN and CP. It is thought to be a newer and efficient form of polymeric carriers and will make the tablet more versatile in its application. | Chitosan, carbopol |
Perioli et al (2009) | A comparison study between batches that includes different type of polymers blended in different ratios. | Chitosan, polyvinyl pyrrolidone, polycarbophil |
Perioli et al (2011) | A comparison study between batches using mucoadhesive polymers alone and in combinations. | Hydroxypropyl methylcellulose, carbopol |
Sánchez et al (2017) | Designed a two-layered vaginal tablet. A comparison study between batches formulated using mixtures with different polymeric ratios. | Sodium carboxymethylcellulose, carbopol, chitosan |
Szymańska et al (2014) | This study formulated vaginal tablets using CHN as a matrix and investigated two different mucosa surrogate as simple adhesive models. | Chitosan, silicified microcrystalline cellulose, sodium stearyl fumarate |
Tunpanich et al (2019) | A comparison study between batches formulated using different types of polymers and their blends in different ratios. | Polycarbophil, hydroxypropyl methylcellulose |
Valenta et al (2001) | A comparison study between batches formulated using non-thiolated and thiolated CHN. The effect of varied amount of TGA on the thiomer was investigated. The study included modification steps and structure characterization methods. | Chitosan, chitosan-thioglycolic acid (thiolated) |
Risk of bias in studies
The risk of bias from the reviewed articles is shown in Table IV. The risk of bias is indicated by symbols; positive “+” indicates that there was risk of bias and negative “–” indicates that there were no/less risk of bias. There was no risk of bias in selection and sampling as this was not applicable to the study design of all the reviewed articles. Performance bias refers to the improved results when a control goes through intervention. This was seen in articles with polymer intervention as formulation using manipulated polymers performed better compared to formulations with non-manipulated polymer. Therefore, there was some risk of bias mostly in articles that included polymer interventions. All reviewed articles were thought to have low risk of both attrition and reporting bias as there were no incomplete outcome data and there were reports on both good and bad batches in the articles, respectively. Some performance assessments were done using self-constructed or modified apparatus setup, thus increasing the risk of measurement bias, as compared to if they were to use a standardized and calibrated apparatus/equipment. Results in all the articles were expressed as mean values ± standard deviation and are indicated in the text. However, some studies conducted further statistical analysis to establish significant differences between groups (formulated batches) compared. These studies have reduced their risk of analysis bias.
Discussion
Mucoadhesive drug delivery systems
There has been extensive research done to circumvent the limitations of discomfort (i.e., messiness, leakage), short stay, and frequent dosing due to insufficient therapeutic dosage of vaginal formulations (3,14,15). Innovative and novel attempts have been made to develop vaginal formulations that consider both the clinical as well as the user’s requirements. Mucoadhesive drug delivery systems exploit the useful property of mucoadhesion of certain biopolymers on interaction with mucus that is present at the targeted physiological sites, for example, the vaginal mucosa (24), though in the absence of goblet cells and the lack of direct release of mucin, the vaginal epithelium is still considered as a mucosal surface (24). Mucin is a glycoprotein that makes up the most part of mucus on mucosal surfaces. Mucin exhibits electrostatic, hydrophobic, and coupling effect, which makes it possible for good adherence of a number of substances to the vaginal mucosa (5). Mucoadhesive polymers that bind to mucin or epithelial surfaces increase the residence time of the dosage form at the action or absorption site, and thus could be useful in solving bioavailability problems resulting from a too short stay of the pharmaceutical dosage form at the absorption site (26).
Author | Sampling Bias | Performance Bias | Attrition Bias | Reporting Bias | Measurement Bias | Bias in Analysis |
---|---|---|---|---|---|---|
Abidin et al (2020) | – | – | – | – | – | – |
Abu El-Enin et al (2020) | – | – | – | – | – | – |
Baki et al (2009) | – | – | – | – | – | + |
Baloglu et al (2011) | – | + | – | – | + | – |
Bartkowiak et al (2018) | – | – | – | – | – | + |
Bhat et al (2010) | – | – | – | – | + | – |
Cazorla-Luna et al (2019) | – | – | – | – | – | – |
Cevher et al (2014) | – | – | – | – | – | – |
Cevher et al (2008) | – | – | – | – | – | – |
El-Kamel et al (2002) | – | – | – | – | + | + |
Fitaihi et al (2017) | – | – | – | – | – | – |
Gupta et al (2013) | – | – | – | – | + | – |
Gök et al (2017) | – | + | – | – | – | + |
Hani et al (2016) | – | – | – | – | + | + |
Hassan et al (2017) | – | – | – | – | + | – |
Hombach et al (2009) | – | + | – | – | – | – |
Kailasam et al (2010) | – | – | – | – | + | + |
Kast et al (2002) | – | + | – | – | – | – |
Khan et al (2017) | – | – | – | – | + | + |
Khan et al (2014) | – | – | – | – | + | + |
Lupo et al (2017) | – | + | – | – | – | – |
Notario-Pérez et al (2019) | – | – | – | – | – | – |
aNotario-Pérez et al (2017) | – | – | – | – | – | – |
bNotario-Pérez et al (2017) | – | – | – | – | – | + |
Nowak et al (2015) | – | + | – | – | + | + |
Pacheco-Quito et al (2020) | – | – | – | – | – | – |
Paczkowska et al (2020) | – | – | – | – | – | + |
Palade et al (2013) | – | – | – | – | – | – |
Patel A. et al (2012) | – | + | – | – | + | – |
Patel A. et al (2011) | – | + | – | – | + | + |
Patel G.M. et al (2010) | – | + | – | – | + | – |
Pendekal et al (2013) | – | – | – | – | + | – |
Pendekal et al (2012) | – | – | – | – | + | + |
Perioli et al (2009) | – | – | – | – | + | + |
Perioli et al (2011) | – | – | – | – | + | + |
Sánchez et al (2017) | – | – | – | – | + | + |
Szymańska et al (2014) | – | – | – | – | – | – |
Tunpanich et al (2019) | – | – | – | – | – | – |
Valenta et al (2001) | – | + | – | – | + | + |
The inclusion of mucoadhesive polymers into vaginal formulations intensifies the contact between formulation and the vaginal mucosa (27,28). Mucoadhesion happens in two stages. Suitable parameters such as wettability, swelling, and hydration of the polymer can ensure close contact between polymer and mucosal layer. This establishment of contact is the first stage of mucoadhesion. Any material can adhere to the mucosa thanks to its viscous nature, but there can be no real adhesion without an interrelation between some specific chemical groups in the polymers and biological tissues, or without establishing an interpenetration of chains (29). Therefore, the second stage of mucoadhesion involves the activation of the polymer in the presence of moisture (hydration), including wetting and swelling of the formulation. The moisture plasticizes the system, which allows the release of mucoadhesive particles and their connection with the mucin by forming van der Waals or hydrogen bonds (5). This then facilitates intimate contact between the formulation and the underlying absorptive surface (14). The main purpose of mucoadhesive drug delivery system is to remain fixed (localization) at the point where the drug’s release and/or absorption can occur (29). This is the great advantage as it prolongs the residence time at the targeted site of application (3,27,30). Hence, the drug’s uptake and bioavailability may be increased, frequency of dosing reduced, and patient compliance improved (24,31). Apart from prolongation of drug release at the site of absorption, drug targeting to the affected site can also be realized (24).
Therefore, the polymers used in these mucoadhesive formulations must be able to adhere to the vaginal mucosa and modulate the drug release from the dosage form. Mucoadhesive polymers should ideally be biocompatible, biodegradable, and non-toxic (11). Commonly employed mucoadhesive polymers can be derived either from synthetic or natural sources (24). Additionally, after hydration, these polymers form hydrophilic matrices that can often be used to produce controlled/sustained-release formulations through their hydration, swelling, and/or erosion (28). Properly designed mucoadhesive vaginal tablets should be able to hydrate and gel very slowly, leading to a prolonged release of the drug to provide a long-term therapeutic effect with improved efficacy, reduced frequency of administration, and minimized drug side effect (32,33).
Vaginal tablets
As mentioned, vaginal dosage forms have been developed and used clinically for many years to deliver gynecological drugs and/or for local therapy of female-related conditions. As shown in Figure 2, the vaginal tablets formulated in all the research articles reviewed is a form of local therapy for female-related conditions. It has been reported that vaginal infections affect nearly 75% of adult women at least once in their lifetime (13). Therefore, it reflects on the research (Tab. II) that has been done with 56% formulated vaginal tablets to treat vaginal infections, including formulations that are antibacterial, antifungal, and antimycotic. There are increasing attention and more recent developments to tackle other female-related conditions such as HIV preventions (15%), vaginal health and management such as hormone therapy (10%), cervical cancer (8%), and preterm labor (3%). About 8% of the research articles reviewed did not specify the application for the vaginal tablet formulated because their research focuses on developing the roles of the carrier in their mucoadhesive vaginal tablets regardless of its therapeutic purpose.
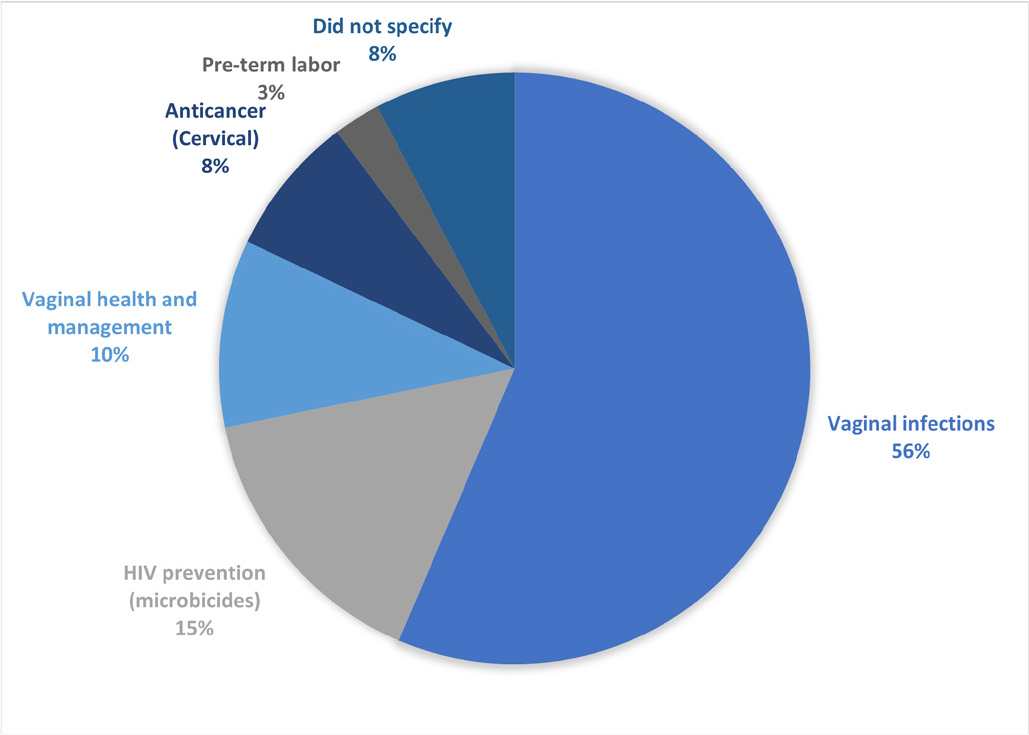
Fig. 2 - Overview of the application for the mucoadhesive vaginal tablets formulated in the 39 research articles reviewed.
Compared to semi-solid systems, solid formulations have the advantage of high dose accuracy and long-term stability (29). Tablets are one of the best means of drug delivery because they are uncomplicated to formulate, and have the feasibility for mass industrial production at a low cost (11,34). Tablets are usually prepared by direct compression, which is an easy, rapid, and cheap method (35). Similar to tablets intended for other administrative routes, vaginal tablets also have advantages including portability, avoidance or antimicrobial agents for preservation, and ease of storage and handling (11,18). Furthermore, the application is convenient, with no applicators or supervision needed, giving the user an added advantage of discretion (18,34).
Tablets are a recurring trend because they permit controlling effects on the drug dissolution. Recently, considerable attention has been paid on novel and controlled release systems to provide a long-term therapeutic concentration of drug following a single administration (36). There has been major advancement in the development of vaginal tablets that increase vaginal residence time and are capable of delivering the active agent for an extended period at a predictable rate using mucoadhesive polymers (3,27,32). It can be an appropriate therapeutic strategy as the required quantity of the drug can be readily administered; with the prolonged residence time, high drug levels at the target site are achievable, simultaneously minimizing unnecessary drug exposure and side effect in other parts of the body (26,32). Mucoadhesive vaginal tablets are relatively easy to insert and do not cause leakages (37). A controlled drug release can be achieved over several hours, if the delivery system does not disintegrate too early (38). In general, the tablet softens and adheres to the vaginal mucosa and is retained in position until dissolution and/or release is complete. They are designed to melt in the vaginal cavity and release the drug for several hours (3). A controlled drug release can be achieved over several hours if the formulation does not disintegrate too early (38). After a short time, the presence of tablet is reported to be no longer noticeable to the patient (3).
Referring to the tablet shapes and designs from the reviewed studies, 80% (31 research articles) are flat-faced cylindrical-shaped tablets, compared to a few (3 research articles) that used an ovoid, concave, and capsule shape and 5 research articles that did not specify the shape of the tablet (Tab. II). However, in these studies the research articles did mention using a disc when testing for the mucoadhesive property of the formulations. The diameters of the tablets range from 15 to 5 mm; 13 mm is the most popular diameter (11 research articles). However, recent research has started using smaller sized tablets. Tablets kept at a smaller size offer a more efficient drug release ability, reduce the risk of an inhomogeneous blend or non-uniform drug content in finished tablets, and can also improve patient comfort and thus compliance (18).
Strategies in formulating mucoadhesive vaginal tablets
The strategies in designing a mucoadhesive vaginal tablet adopted by the reviewed articles are divided into six main categories (Fig. 3). The strategies used are listed as follows: (i) polymer blends (46%), (ii) thiomers (18%), (iii) intermolecular complexes (18%), (iv) physical design (10%), (v) micro-particulate technology (5%), and (vi) formulation technique (3%). The percentages are the number of research articles that used the design strategy respectively. The categories were decided by the reviewers based on the theoretical application/theme used in designing the tablets. As the advancement of mucoadhesive drug system continues, there is a vast number of novel strategies that are being invented and it can get quite competitive. Therefore, this review focuses greatly on the assessment methods that are conducted by the research articles reviewed, to evaluate the degree of mucoadhesion for vaginal tablet formulation.
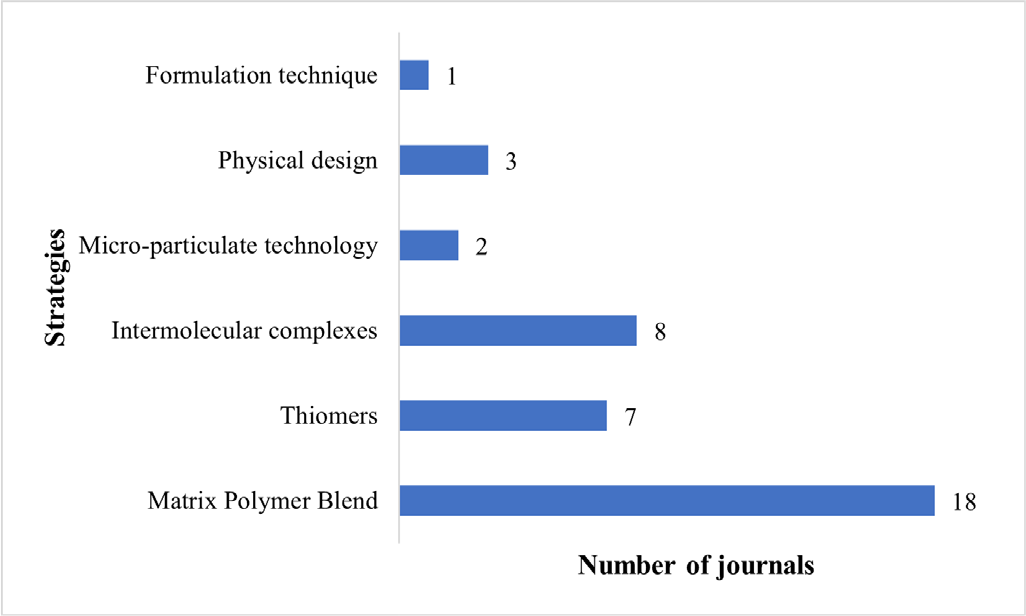
Fig. 3 - The main categories of the strategies adopted in the research articles reviewed in designing their mucoadhesive vaginal tablets.
Physical evaluations of tablets/physicochemical properties
Tablet formulations are evaluated to comply to specific pharmacopoeia requirements, including precompression (e.g. tabletability, flowability), tablet uniformity, friability, disintegration time, tablet ejection force, and dissolution performance and any other relevant evaluations (18). If any of these requirements are not met, the formulation should be excluded and new formulation is designed and re-evaluated to address identified deficiencies (18).
Precompression evaluation investigates the powdered mixtures’ compressibility profile or matrix granules before compression. The flowability determines the mechanical behavior of the powders, which can affect the tablets’ weight, hardness, and content uniformity (39). For example, powder flowability can determine the ease with which the powder can be fed into the die and non-flowing powders can also cause the non-uniformity incorporation of drug compounds in the powders (40). Therefore, flow properties of pharmaceutical powders are critical to manufacturing. There are various methods that can be used as an indication of powder flowability, including measurement of angle of repose, bulk density, tapped density, and Carr’s compressibility index (CI) or Hausner ratio (HR) (39). Angle of repose (°) is the steepest slope of the unconfined material, measured from a horizontal plane (41). Repose angles are reported within the range of 30°–55°. It is described that less than 30° are powders that has high flowability and more than 55° are non-flowing powders (41). Bulk and tapped densities are commonly reported together, as it measures the volume occupied by the powder (of known mass), g/mL, in a graduated cylinder mounted onto a tapping platform. The volume occupied before tapping is reported as the bulk density and after a range of 250–1,000 taps, the volume obtained is reported as the tapped density (18). Both these densities are used to calculate CI and HR to characterize flowability. CI uses a percentage scale of 0–100, where 0–10% indicates excellent flowability. HR has a range of 1.00–1.50, where 1.00–1.11 indicates excellent flowability (18,40). Poor flowability of powders are indicated with >31 CI and >1.60 HR. Any value in between can describe the powder’s flowability as good, fair, and passable. These evaluations are to some extent interrelated to one another and help to describe the particles’ flow and how readily the material undergoes a change in volume when compressed (18,32).
Good compressibility, on the other hand, describes a material capable of achieving desired tablet hardness at low compression pressure. It is important to remember that any unnecessary increase in compression pressure can induce physical changes upon the compressed material (37). Therefore, careful selection of optimal compression pressure to be employed in manufacturing the tablets is important. A pressure will not physically damage the material and can produce tablets with good tensile strength.
Figure 4 shows the list of the types of tablet evaluations that have been conducted by the research articles reviewed (Tab. V). About 54% and 44% of the research articles conducted the hardness and friability test, respectively. These are considered fundamental evaluations as it ensures that the formulations have satisfactory tablet strength to withstand abrasion or chipping during packaging, handling, and shipping (18,26). Evaluations of tablet’s hardness typically involve crushing actions of individual tablets and the crushing force used can be reported in Newtons (N) and/or kilograms (kg). The crushing force reported serves as an information to avoid crushing the tablets during manufacturing and packaging processes. Friability evaluation involves subjecting tablets in circular motions and the result is reported as the weight difference of the tablets before and after the test, in percentage. Weight loss of below 1% is ideal. The friability test can help indicate the brittleness of the tablets, which helps to prevent chipping and abrasion of the tablets (18).
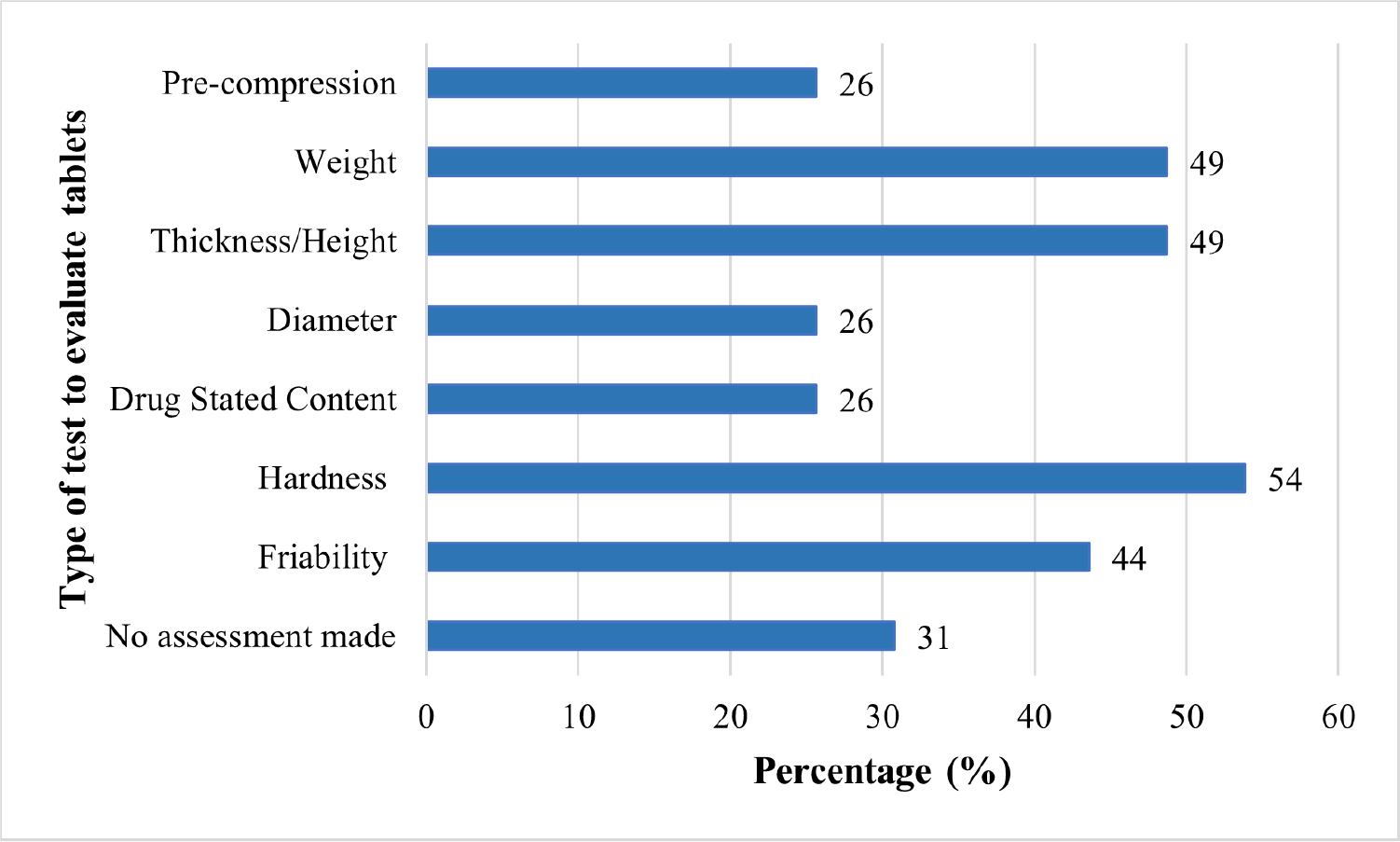
Fig. 4 - This bar chart shows the type and frequency of the tablet evaluations conducted within the research articles reviewed.
Author | Precompression | Uniformity measurement | Durability measurement | ||||
---|---|---|---|---|---|---|---|
Weight | Height/thickness | Diameter | Drug content | Hardness | Friability | ||
Abidin et al (2020) | √ | √ | √ | √ | √ | √ | √ |
Abu El-Enin et al (2020) | – | √ | – | – | √ | √ | √ |
Baki et al (2009) | √ | – | √ | √ | – | – | √ |
Baloglu et al (2011) | – | √ | √ | √ | – | √ | √ |
Bartkowiak et al (2018) | – | – | – | – | – | – | – |
Bhat et al (2010) | – | √ | – | – | √ | √ | √ |
Cazorla-Luna et al (2019) | – | √ | √ | √ | – | √ | – |
Cevher et al (2014) | – | √ | – | – | – | √ | √ |
Cevher et al (2008) | – | – | – | – | – | – | – |
El-Kamel et al (2002) | – | – | – | – | √ | – | – |
Fitaihi et al (2017) | √ | √ | √ | – | √ | √ | √ |
Gupta et al (2013) | – | √ | √ | – | √ | √ | √ |
Gök et al (2017) | – | – | – | – | – | – | – |
Hani et al (2016) | – | √ | √ | – | √ | √ | √ |
Hassan et al (2017) | – | √ | √ | – | √ | √ | √ |
Hombach et al (2009) | – | – | – | – | – | – | – |
Kailasam et al (2010) | – | – | – | – | – | – | – |
Kast et al (2002) | – | – | – | – | – | – | – |
Khan et al (2017) | √ | √ | √ | √ | – | √ | √ |
Khan et al (2014) | √ | √ | √ | √ | – | √ | √ |
Lupo et al (2017) | – | – | – | – | – | – | – |
Notario-Pérez et al (2019) | – | – | – | – | – | – | – |
aNotario-Pérez et al (2017) | – | – | – | – | – | – | – |
bNotario-Pérez et al (2017) | – | – | – | – | – | – | – |
Nowak et al (2015) | – | – | – | – | – | – | – |
Pacheco-Quito et al (2020) | – | √ | √ | √ | – | – | – |
Paczkowska et al (2020) | – | √ | √ | √ | – | √ | – |
Palade et al (2013) | – | – | – | – | – | – | – |
Patel A. et al (2012) | – | √ | √ | – | – | √ | – |
Patel A. et al (2011) | – | – | – | – | – | √ | √ |
Patel G.M. et al (2010) | – | √ | √ | – | – | √ | – |
Pendekal et al (2013) | – | – | – | – | – | – | – |
Pendekal et al (2012) | – | – | – | – | – | – | – |
Perioli et al (2009) | – | – | √ | – | – | √ | √ |
Perioli et al (2011) | – | – | √ | – | – | √ | √ |
Sánchez et al (2017) | – | √ | √ | √ | – | √ | – |
Szymańska et al (2014) | – | √ | √ | √ | √ | √ | √ |
Tunpanich et al (2019) | √ | √ | √ | – | √ | √ | √ |
Valenta et al (2001) | – | – | – | – | – | – | – |
Weight, thickness/height, diameter, and drug content measurements are evaluations of uniformity in the tablets manufactured. The evaluations of tablet uniformity are typically reported as an average and standard deviation. Weight, thickness/height, and diameter of any individual tablets should not deviate more than 5% from the average measurement. Drug content within 95–105% from the expected content is considered acceptable (18). The tablet uniformity is important as it is an indication of a consistent tablet formulation and manufacturing process (26). Furthermore, uniformed tablets can also contribute to consistent tablet performance.
Swelling assessment
Mucoadhesive drug delivery systems require mucoadhesive polymers that can adhere to the vaginal mucosa and swell rapidly in aqueous environmental conditions (36). Some studies have suggested that tablet swelling is an important parameter to be studied before considering mucoadhesion (35). The swelling characteristic of a polymer contributes to their adhesive capacity and in order to manifest maximum adhesive strength, an optimum water uptake (hydration) is needed for the polymer particles (26). However, if the hydration is too high, the adhesion property is expected to be reduced due to the competition between water molecules and the active groups in the mucin chains of the vaginal mucosa to bind to the functional groups of the polymer (18). Therefore, the swelling property (degree of hydration) should be investigated and considered when designing the mucoadhesive vaginal tablet formulations. About 90% of the vaginal tablets in the research articles reviewed were subjected to swelling tests and the results were used to consider the tablet size and improve drug retention and drug release kinetics.
The swelling characteristic is typically done by subjecting the tablet to hydration in an aqueous solvent used in dissolution or disintegration studies. The degree of hydration may be reported using units, including swelling index (SI), swelling ratio (SR/Q), water uptake ratio (WUR), mass swelling factor (MSF), swelling degree (Qe), water uptake (WU), swelling percentage, hydration percentage, matrix erosion (ME), and matric dissolution (DS). Although there are various units to report the numerical data of swelling, all of these measure the degree of hydration by the change in weight that occurs in the tablet formulations after hydration. Therefore, it is essential to record the weight of individual tablets prior to hydration. Positive values indicate that the percentage of swelling or weight gain is greater than the initial weight of the dry formulation, while negative values indicate the weight is less than the dry formulations and this can be due to erosion or dissolution of the system in the solvent (11).
From the research articles reviewed, the swelling test was done using three different methods: (i) immersion (49%), (ii) gravimetric (31%), and (iii) water absorbing method (10%) (Tab. VI). The different method describes the different setting in hydrating the tablets. The immersion method involves total submergence of the tablet into a solvent. Gravimetric method involves fixing the tablet to a needle and suspending it into a solvent. This method is also called the tea bag method in some research articles. There was no specific volume; from the research articles reviewed there was a range of volume from 5 mL up to 1000 mL of solvent used for these methods. In the third method, individual vaginal tablet is placed on 2% agar gel plates to assess the water absorbing capacity of each tablet. Most of these swelling methods were conducted in incubators or water bath, kept at 37 ± 1°C, and some remained stationary or was put in a shaking motion. Two research articles reported to conduct the swelling test in ambient temperature (14,42). Individual tablets are taken out at scheduled time intervals and excess surface water was carefully removed. The swollen tablets were then reweighed for the swelling assessment. Proper swelling characteristic will contribute to effective mucoadhesion and controlled release of the drug (14).
Author | Method | Shaking motion | Incubation temperature (°C) | Reporting unit | Swelling witness test | ||
---|---|---|---|---|---|---|---|
Immersion | Gravimetric | Water absorbing | |||||
Abidin et al (2020) | √ | – | 37 ± 1 | Swelling index (SI) | – | ||
Abu El-Enin et al (2020) | √ | – | Ambient | Swelling index (SI) | – | ||
Baki et al (2009) | – | – | – | – | 37 ± 1 | – | – |
Baloglu et al (2011) | √ | – | 37 ± 1 | Water uptake ratio (WUR) | – | ||
Bartkowiak et al (2018) | √ | – | 37 ± 1 | Mass swelling factor (MSF) | – | ||
Bhat et al (2010) | √ | – | 37 ± 1 | Swelling index (SI) | – | ||
Cazorla-Luna et al (2019) | √ | √ | 37 ± 1 | Swelling ratio (SR/Q) | √ | ||
Cevher et al (2014) | √ | – | 37 ± 1 | Swelling index (SI) | – | ||
Cevher et al (2008) | √ | – | 37 ± 1 | Swelling index and Matrix erosion (ME) | – | ||
El-Kamel et al (2002) | √ | – | 37 ± 1 | Swelling index (SI) | – | ||
Fitaihi et al (2017) | √ | – | 37 ± 1 | Swelling percentage (%S) | – | ||
Gupta et al (2013) | √ | – | 37 ± 1 | Reweighed | – | ||
Gök et al (2017) | √ | – | 37 ± 1 | Swelling degree (Qe) | – | ||
Hani et al (2016) | √ | – | 37 ± 1 | Percentage of hydration | – | ||
Hassan et al (2017) | √ | – | 37 ± 1 | Swelling index (SI) | – | ||
Hombach et al (2009) | √ | – | 37 ± 1 | Reweighed | – | ||
Kailasam et al (2010) | √ | – | 37 ± 1 | Swelling index (SI) | – | ||
Kast et al (2002) | √ | – | 37 ± 1 | Reweighed | – | ||
Khan et al (2017) | √ | – | Ambient | Swelling index (SI) | – | ||
Khan et al (2014) | √ | – | 37 ± 1 | Swelling index (SI) | – | ||
Lupo et al (2017) | √ | – | 37 ± 1 | Water uptake (WU) | – | ||
Notario-Pérez et al (2019) | √ | √ | 37 ± 1 | Swelling ratio (SR) | – | ||
aNotario-Pérez et al (2017) | √ | √ | 37 ± 1 | Swelling ratio (SR) | √ | ||
bNotario-Pérez et al (2017) | √ | √ | 37 ± 1 | Swelling ratio (SR) | √ | ||
Nowak et al (2015) | √ | – | 37 ± 1 | Water uptake percentage | – | ||
Pacheco-Quito et al (2020) | √ | √ | 37 ± 1 | Swelling ratio (SR) | √ | ||
Paczkowska et al (2020) | – | – | – | – | 37 ± 1 | – | – |
Palade et al (2013) | – | – | – | – | 37 ± 1 | – | – |
Patel A. et al (2012) | √ | – | 37 ± 1 | Swelling percentage (%S) | – | ||
Patel A. et al (2011) | √ | – | 37 ± 1 | Swelling percentage (%S) | – | ||
Patel G.M. et al (2010) | √ | – | 37 ± 1 | Swelling percentage (%S) | – | ||
Pendekal et al (2013) | √ | – | 37 ± 1 | Swelling index (SI) | – | ||
Pendekal et al (2012) | √ | – | 37 ± 1 | Swelling index (SI) | – | ||
Perioli et al (2009) | √ | – | 37 ± 1 | Hydration percentage and Matrix erosion (ME) | – | ||
Perioli et al (2011) | √ | √ | 37 ± 1 | Hydration percentage and Matrix erosion (ME) | – | ||
Sánchez et al (2017) | √ | – | 37 ± 1 | Hydration percentage and Matrix dissolution (DS) | – | ||
Szymańska et al (2014) | √ | – | 37 ± 1 | Swelling index (SI) | – | ||
Tunpanich et al (2019) | √ | – | 37 ± 1 | Swelling index (SI) and Matrix erosion (ME) | – | ||
Valenta et al (2001) | – | – | – | – | 37 ± 1 | – | – |
The polymer will start to swell upon hydration, and a viscous gel layer should start to form around the tablet core (26). As it reaches the maximum swelling capacity, erosion occurs until complete dissolving or erosion of tablets (14). The formation of this gel layer has been regarded as an essential first step that would govern the drug release from the vaginal tablet formulation (26).
Swelling witness test
In the past few years, researchers have started to include a complementary technique using prepared swelling witnesses to better understand the swelling behavior of the mucoadhesive polymers used and structural characterization of the tablets (16,43). It is a method that evaluates the tablet’s capacity to absorb water or determine the entry pattern of the solvent into the tablet during the hydration process (16). The mobility (movement) of the solvent within the tablet formulation controls the swelling and erosion of the tablets, thus it plays a role in the drug release as well (11).
Tablet formulations were left to hydrate and swell in a chosen solvent in accordance with predetermined time and/or until it reaches the maximum swelling capacity. The swollen tablets were then immediately lyophilized (freeze-dried). Water is removed during freeze-drying, and the space that was originally occupied by the solvent is transformed into pores (hollow gaps), obtaining porous structures similar to a sponge, that are now called swelling witnesses (16,43). The swelling witnesses can then be observed and analyzed by field emission scanning electron microscopy (SEM) (11,16). Corresponding SEM micrographs shows the witness’ microstructures which vary depending on the type of polymer and different formulations. Mercury porosimetry can be used to determine the pore size distributions (PSDs) of the witnesses, which reflects the pores (gaps/hollows) that were occupied by the solvent before the freeze-drying process (16). The gaps inside the polymers are related to the swelling ratio, as the gaps get larger the higher its ability to capture water, and the tablet formulation swells more (43). Polymers with good swelling capacity will produce a narrow PSD (the pores are closer together) with high pore sizes. In contrast, polymers with minimal swelling properties produce a wider PSD (the pores are further apart) and smaller pore sizes. The wider PSDs, however, do have an appealing advantage, where it will help the tablet to maintain the shape while the drug diffuses slowly between them (43). Furthermore, formulations capturing less water are expected to be more comfortable for the user.
There were four research articles that included the swelling witness in their research, and they have reported varying microstructures in their witnesses depending on the nature of their polymers and the changes in the swelling witness in different formulations (11,16,29,43). When a solvent enters the polymer during swelling, it creates different microstructures depending on the nature of the polymer. Figure 5 is a compilation of SEM micrographs from the research articles that depict the most distinctive microstructures corresponding to the type of drug release that it can achieve. Figure 5A shows a channeled microstructure (elongated channels) allowing gradual uptake of the surrounding solvent which translates into a moderate swelling behavior (29). The moderate swelling can help maintain the shape of the tablet while the drug diffuses slowly between them (43). Figure 5B shows a sponge-like microstructure with numerous pores, which the solvent can circulate with some difficulty, which would also result in moderate swelling capacity of the formulation (11). Figure 5C shows a microstructure arranged in parallel sheets with the absorbed solvent between them (29). Formulations using this type of polymer will swell the most and can remain swollen the longest, as there is a high capacity for very effectively retaining water between the sheets. However, although the water cannot escape, the drug is able to diffuse through the polymer sheets, thus reducing their ability to retain the drug longer (43). Figure 5D is included to show one of the examples when a formulation was unable to swell (29). This formulation showed a grainy microstructure with different-sized particles and was reported to have failed in controlling drug delivery (43). These images are compiled from research articles reviewed and reproduced from references (11) and (29) under the Creative Common Attributions License.

Fig. 5 - Electron microscopy micrographs of distinct swollen witness in different polymers compiled from four research articles reviewed. The different panels show the different type of microstructures observed: A) channeled microstructure (250 μm) (29); B) sponge-like microstructure (1 mm) (11); C) parallel sheets microstructure (250 μm) (29); and D) a material that is unable to swell (250 μm) (29). (Pictures are reproduced from references (11) and (29) under the Creative Common Attributions License.)
This assessment helps to identify the effects or modification in the microstructure arrangement, WU process, and swelling capability, when different polymers are mixed and/or drugs are incorporated into the formulation. The presence of other materials that do not swell can make it difficult for the polymer to swell as usual. In some cases, materials that do not swell can clog the pores, thus reducing the sizes of the pores or hindering the drug diffusion through the polymer (43). Therefore, WU rates are reduced and can be the cause for slower drug release from the tablet formulations (16). Although the key criteria for selecting the optimum formulation are control over the drug release and mucoadhesion to the vaginal mucosa, the amount of swelling must be taken into account in formulations that can achieve these criteria but capturing less water, as it will be more comfortable (11).
Drug release assessment
The terms “drug dissolution” and “drug release” are not synonyms, although they are often not appropriately distinguished. Drug dissolution refers to the process of diffusion of the drug into the solvent. Unless the drug dissolution process is the factor that can manipulate the release of the drug, the drug dissolution and drug release can be considered synonyms. In all other cases, the drug release is the more appropriate term. Upon contact with the dissolution solvent, water penetrates the tablet formulation and can dissolve the drug content. The dissolved drug substance subsequently diffuses from the tablet due to the concentration gradient. Additionally, the tablet formulation might also undergo several changes including swelling and consequent dissolution in the solvent, all contributing to the overall drug release process (44). Assessing drug dissolution/release from the tablet formulation is extremely important within the absorption process to be effective and it is indicative of the tablet’s potential in vivo performance and clinical applications (44,45). Ideally, 100% of drug dissolution is targeted in all formulations as it can increase bioavailability and the efficacy of the formulation. However, it depends on the objective of the therapy to have an immediate or controlled/extended drug release. A constant drug release rate over the targeted time also contributes to the formulation performance (18).
The in vitro dissolution/release test represents an important tool for this purpose as it can be used to assess the dissolution and release profile of the formulation in an artificial vaginal environment (45). Drug releases are primarily recorded at predetermined time intervals to observe the difference in drug concentration over time. It has become an important tool in the drug product development phase and its quality control and the regulatory approval process (44). For many types of mucosal formulations including vaginal tablets, drug testing is performed using the apparatus developed for oral formulations (44). Today four apparatuses for dissolution testing of solid dosage forms are described in pharmacopoeias: paddle apparatus, basket apparatus, reciprocating cylinder, and flow through cell. From the research articles reviewed (Tab. VII), 46% have used the paddle apparatus and 26% used the basket method, and others have used alternative dissolution apparatus. Most methods used are designed to mimic the general conditions encountered in the physiological environment of the vagina, including the pH of the dissolution solvent and maintaining a temperature of 37 ± 1°C during testing. A total of 72% from the reviewed studies have specified the pH of the dissolution medium used and the mean pH was 4.7, which is within the range of the pH of the vaginal fluid (pH 4–5) (46,47).
Author | Novel setup/other | Dissolution medium | pH | Volume (mL) | Sink conditions | RPM | USP paddle | USP basket | Shaking water bath | Still water bath | Disintegration test |
---|---|---|---|---|---|---|---|---|---|---|---|
Abidin et al (2020) | 2% sodium dodecyl sulfate aqueous solution | 4.2 | 900 | √ | 100 | √ | X | ||||
Abu El-Enin et al (2020) | Simulated vaginal fluid | 4.5 | 100 | √ | 50 | √ | X | ||||
Baki et al (2009) | Phosphate buffer | 4.6 | 4 | X | X | √ | X | ||||
Baloglu et al (2011) | √ | Simulated vaginal fluid | X | 6 | X | √ | |||||
Bartkowiak et al (2018) | Simulated vaginal fluid | 5.8 | 500 | √ | 50 | √ | X | ||||
Bhat et al (2010) | 100 mM acetate buffer | 6 | 500 | √ | 25 | √ | X | ||||
Cazorla-Luna et al (2019) | Simulated vaginal fluid | X | 80 | √ | 15 | √ | X | ||||
Cevher et al (2014) | Lactate buffer | 5 | 250 | X | 75 | √ | X | ||||
Cevher et al (2008) | Lactate buffer | 5 | 500 | √ | 75 | √ | X | ||||
El-Kamel et al (2002) | Citrate buffer | 5.5 | 650 | √ | 25 | √ | X | ||||
Fitaihi et al (2017) | McIlvaine’s citrate buffer | 4.8 | 250 | √ | 50 | √ | X | ||||
Gupta et al (2013) | Citrate phosphate buffer | 4.5 | 600 | √ | 100 | √ | X | ||||
Gök et al (2017) | Lactate buffer | X | 500 | X | 75 | √ | X | ||||
Hani et al (2016) | Simulated vaginal fluid | X | 900 | √ | 100 | √ | X | ||||
Hassan et al (2017) | Citrate buffer | 4.5 | 900 | √ | 50 | √ | X | ||||
Hombach et al (2009) | Simulated vaginal fluid | 4.2 | 500 | √ | 20 | √ | X | ||||
Kailasam et al (2010) | 1 M phosphate buffer | 4.0 | X | X | 50 | √ | X | ||||
Kast et al (2002) | 100 mM acetate buffer | 6.0 | 4 | √ | 100 | √ | √ | ||||
Khan et al (2017) | Simulated vaginal fluid | 4.2 | 100 | √ | 50 | √ | X | ||||
Khan et al (2014) | Simulated vaginal fluid | 4.2 | 100 | √ | 50 | √ | X | ||||
Lupo et al (2017) | √ | Simulated vaginal fluid | 4.2 | 6 | √ | 630 | √ | ||||
Notario-Pérez et al (2019) | Simulated vaginal fluid | X | 80 | √ | 15 | √ | X | ||||
aNotario-Pérez et al (2017) | Simulated vaginal fluid | X | 80 | X | 15 | √ | X | ||||
bNotario-Pérez et al (2017) | Simulated vaginal fluid | X | 80 | √ | 15 | √ | X | ||||
Nowak et al (2015 | – | – | – | – | – | – | – | – | – | √ | |
Pacheco-Quito et al (2020) | Simulated vaginal fluid | X | 80 | √ | 15 | √ | X | ||||
Paczkowska et al (2020) | Phosphate buffer | 4.5 | 150 | √ | 50 | √ | X | ||||
Palade et al (2013) | Acetate buffer | 4.2 | 900 | √ | 60 | √ | X | ||||
Patel A. et al (2012) | Phosphate buffer | 4.0 | 500 | √ | 30 | √ | X | ||||
Patel A. et al (2011) | Phosphate buffer | 4.0 | 500 | √ | 30 | √ | X | ||||
Patel G.M. et al (2010) | Citrate buffer | 4.0 | 500 | √ | 50 | √ | X | ||||
Pendekal et al (2013) | Simulated vaginal fluid | 4.2 | 500 | √ | 50 | √ | X | ||||
Pendekal et al (2012) | Simulated vaginal fluid | 4.2 | 500 | √ | 50 | √ | X | ||||
Perioli et al (2009) | Simulated vaginal fluid | X | 900 | √ | 100 | √ | X | ||||
Perioli et al (2011) | Simulated vaginal fluid | X | X | √ | 100 | √ | X | ||||
Sánchez et al (2017) | Simulated vaginal fluid | 5.5 | 600 | √ | 100 | √ | √ | ||||
Szymańska et al (2014) | 0.08 M acetic buffer | 4.5 | 900 | √ | 75 | √ | X | ||||
Tunpanich et al (2019) | 1% sodium dodecyl sulfate aqueous solution | 5.5 | 900 | √ | 75 | √ | X | ||||
Valenta et al (2001) | 100 mM phosphate buffer | 6.0 | 20 | √ | 100 | √ | X |
*NB: X = did not specify; “–“ = was not conducted.
Although it is acceptable for research articles to use the apparatus mentioned, it should be pointed out that these methods utilize a large volume of dissolution solvent and the rotational movement (36,44). These are generally far from the real in vivo conditions at the vaginal mucosa, thus the method used would not have given an accurate information. Some articles have taken the initiative to use smaller-volume apparatus, and some have designed a novel drug release technique that considers the correct amount of vaginal fluid and its turnover in the vaginal lumen. As reviewed (Tab. VII), 59% of the studies used larger dissolution solvent volumes ranging from 150 to 900 mL; 23% have tried reducing the volume, using 20–100 mL of dissolution solvent; however, even these volumes are significantly higher than those available to the mucoadhesive vaginal tablet on the vaginal mucosa (44). The daily production of vaginal fluid is approximately 6 mL and 0.5–0.75 mL is continually present in the vagina (36). Only 10% of the studies have considered using the correct estimated volumes ranging from 4 to 7 mL to closely mimic the in vivo conditions for vaginal administration. As in the case of the rotational movement, there is no specification. Most studies have chosen a setting of revolutions per minute (rpm) that is appropriate only to their tablet formulations. However, 100 and 50 rpm are commonly used. The rpm used in the research articles reviewed ranges from 15 to 100 rpm and one study (48) used an astounding 630 rpm as its dissolution technique employs a thermomixer. Even though some methods do not represent the correct vaginal environment, the results of these evaluations can still contribute to preliminary findings of the formulation.
An increasing number of research have modified and designed drug release techniques that can better simulate the specific conditions of the vaginal environment for mucoadhesive vaginal tablets. Various parameters must be considered when designing a dissolution apparatus, including selection of apparatus, volume and composition of the dissolution medium, environmental conditions of the absorption site (e.g., agitation), and surface exposure of the tablet (36,44). However, as new technique emerges, there is a need now to further validate and standardize the methods developed (44). For further advancement of drug release techniques, more physiological vaginal conditions should be considered. For instance, different conditions occur during menstrual cycle or at different ages, along with the enzymatic activity of the vaginal microflora. In particular, the pH and composition of vaginal fluid change from low pH values (3.5–4.5) during the ovulation phase to a higher pH during menstruation (49).
Novel release study method by Baloglu et al (36)
In this study, a new simple technique mimicking the vaginal environment was developed to investigate the release behavior of the vaginal tablet formulation. The apparatus mainly consists of a perfusor and syringe which are connected with a thin latex connector and a sample collection vessel as illustrated in Figure 6. (Pictures are reproduced from (36) and have received copyrights permission by the publisher on April 28, 2022.) The syringe used (without needle) has an internal diameter of 20 mm and total length of 75 mm to simulate the vaginal physiology. Tablets were placed at the bottom of the syringe and the assembly was dipped into a water at 37 ± 0.5°C. A perfusor was connected to the top of the syringe to supply a total of 6 mL of vaginal fluid to the tablets in 24 hours. The same amount of sample was collected concurrently from the bottom (36).
Kinetic analysis
To understand the mechanism of drug release from mucoadhesive tablets, some research articles have plotted the in vitro drug release data in kinetic equation models. Many model-dependent approaches can be used to investigate the best-fit parameters (32). The common equation used in most kinetic studies of the research articles reviewed is the Korsmeyer-Peppas semi-empirical model (Eq. [1]),
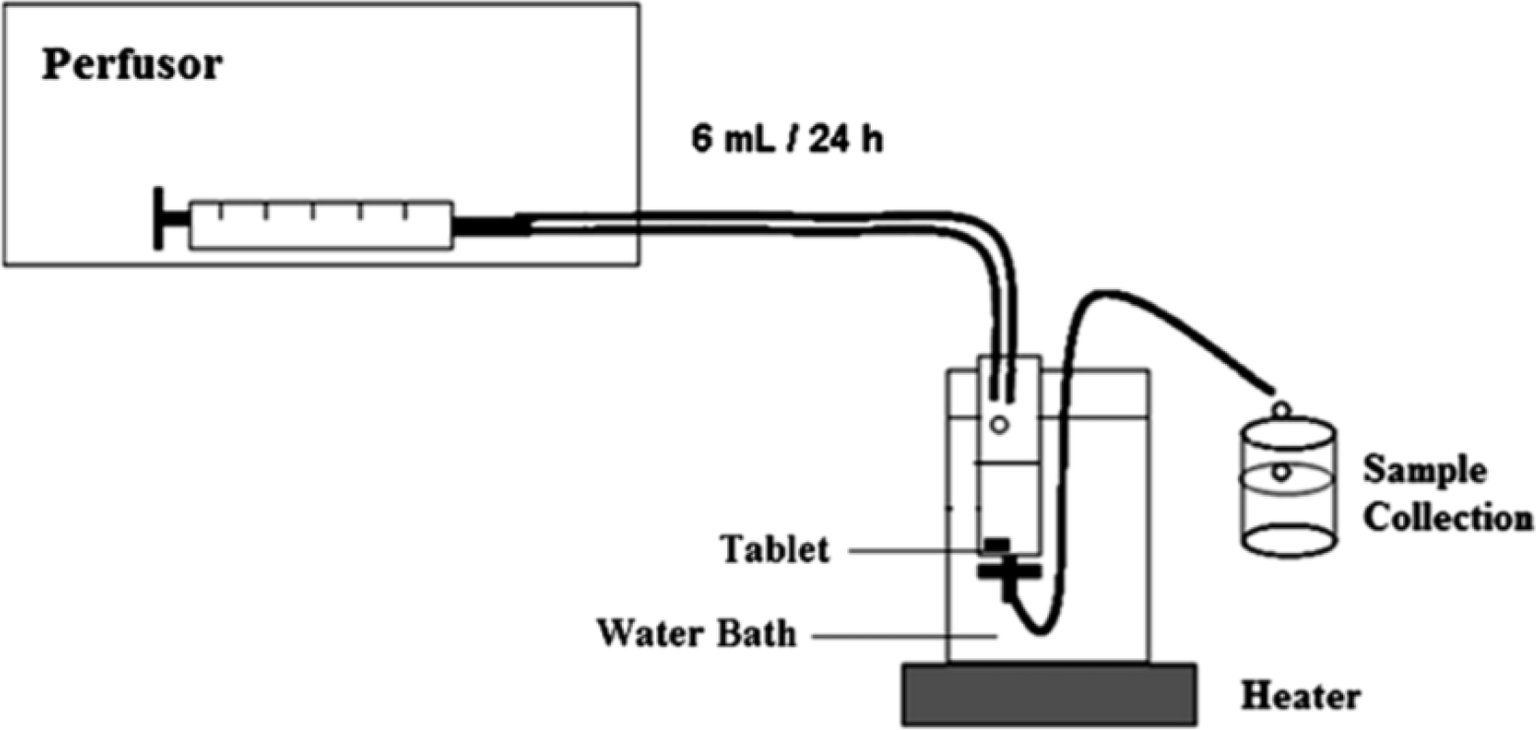
Fig. 6 - Schematic drawing of the in vitro release studies developed by Baloglu et al. (36). (Picture was taken from reference (36) and has received copyrights permission by the publisher on April 28, 2022.)
Equation 1
where Mt/M∞ is the fractional amount of drug release at time t, kKP is the release rate constant, and n is the diffusional exponent that characterizes the type of release mechanism used during the dissolution process (26). The values n and kKP were estimated using a linear regression of log (Mt/M∞) compared with log t. For the case of cylindrical tablets, n = 0.45 corresponds to a Fickian diffusion release (Case I diffusional), 0.45 < n < 0.89 to a non-Fickian or anomalous diffusion, n = 0.89 to a Case-II transport or typical zero-order release and n > 0.89 to a super Case-II transport (13). Most research articles have obtained diffusional exponent that indicates a non-Fickian or anomalous diffusion, which involves a combination of both diffusion and erosion mechanism (13,26).
There are other types of kinetic release models including Higuchi (32), Weibull (32), Hopfenberg (11), Hixson and Crowell (11), and Moore and Flanner (37) that can be used; however, as mentioned the Korsmeyer-Peppas kinetic model is the most relevant and commonly used.
Mucoadhesion assessment
Defining the mucoadhesive characteristic of the vaginal tablet formulation is of great importance to prevent a decrease in the detachment of the tablet from the vaginal mucosa, prolonging the residence time of the formulation at the site of administration (36). As drug diffuses from the gel layer of the mucoadhesive polymer to the mucus and absorbed by the tissue lining the vaginal cavity, it is essential to quantify the interaction between the mucoadhesive formulation and the vaginal mucosal surface (25,36). This is reflected in Figure 7, showing only 8% of the research articles did not conduct any mucoadhesion assessment.
The main feature of mucoadhesion is the tablet formulation’s attachment strength (tensile strength) to the vaginal mucosa. There are numerous tests proposed and adopted in the research articles reviewed to determine the attachment strength. The test methods reviewed can be divided into two major categories: (i) in vitro/ex vivo methods and (ii) in vivo methods. It can be summarized from the research articles reviewed (Fig. 7 and Tab. VIII) that 33% are exclusive to only one type of in vitro/ex vivo assessment method, 41% have used multiple in vitro/ex vivo methods and 18% have assessed the mucoadhesion strength in combination of both in vitro/ex vivo and in vivo methods. The in vitro/ex vivo method is by far the most common as in vivo mucoadhesive studies are costly, time consuming, and ethically sensitive (3). However, when a study does include in vivo mucoadhesive studies, it would normally only involve assessment of the most optimal vaginal tablet formulation.
In vitro/ex vivo mucoadhesive assessment
The in vitro/ex vivo methods can be further divided into forced detachment and residence time methods. In conducting these tests, it is recommended to also test blank formulations and compare it to the drug formulations. It can be useful in confirming and establishing the mucoadhesive properties of the chosen polymers or polymer blends (16). It can also be a control to evaluate if the mucoadhesive properties are altered after the addition of the drug substance with the polymers.
Forced detachment method
This in vitro/ex vivo method is based on measuring the force required for destructing the adhesive bond between the vaginal tablet and the vaginal tissue. It involves a physical act of pulling apart the vaginal tablet from the tissue, representing complete detachment (32). This can be done using a tensile tester (e.g., TA-XTplus), where the vaginal tissue is fixed onto the lower clamp/plate and the vaginal tablet can be fixed onto the upper clamp/probe of the tensile tester (16). The vaginal tablet should be wetted with the dissolution medium, then both the tablet and tissue are brought into contact with a slight contact force for a minimum of 30 seconds to allow the formation of the adhesive (13). Next the tablet and the mucosa were pulled apart at a constant speed until complete detachment and the force applied to pull them apart is recorded (14,23,32). There are a total of 31 research articles that have conducted this type of assessment method; 13 research articles have used a tensile tester and the remaining 18 research articles have devised a simple apparatus or have used other alternatives, however, still in keeping with the basic principle of this assessment method.
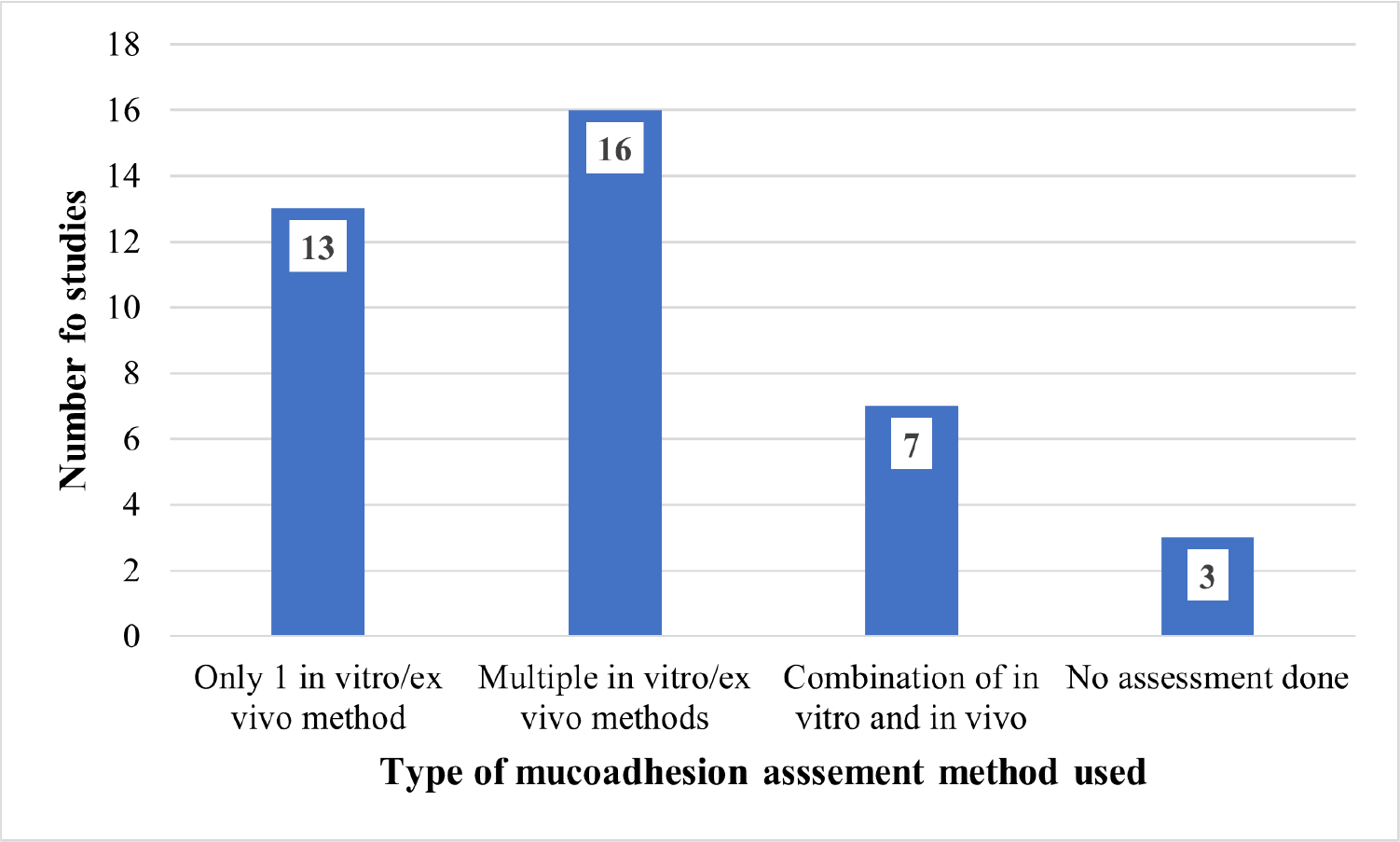
Fig. 7 - Summary of the type of methods in the research articles reviewed in assessing the mucoadhesive property of their vaginal tablet formulations.
Author | Tensile method to measure force | Methods to measure over time | In vivo | |||
---|---|---|---|---|---|---|
TA-XT | Novel/modified | Dissolution apparatus | Wash-off/rinsing method | Immersion/submerged | ||
Abidin et al (2020) | √ | |||||
Abu El-Enin et al (2020) | √ | √ | √ | |||
Baki et al (2009) | – | – | – | – | – | – |
Baloglu et al (2011) | √ | √ | ||||
Bartkowiak et al (2018) | – | – | – | – | – | – |
Bhat et al (2010) | √ | |||||
Cazorla-Luna et al (2019) | √ | √ | ||||
Cevher et al (2014) | √ | |||||
Cevher et al (2008) | √ | |||||
El-Kamel et al (2002) | √ | |||||
Fitaihi et al (2017) | √ | √ | ||||
Gupta et al (2013) | √ | √ | ||||
Gök et al (2017) | √ | √ | ||||
Hani et al (2016) | √ | √ | √ | |||
Hassan et al (2017) | √ | √ | √ | |||
Hombach et al (2009) | √ | √ | ||||
Kailasam et al (2010) | √ | |||||
Kast et al (2002) | √ | |||||
Khan et al (2017) | √ | √ | ||||
Khan et al (2014) | √ | √ | ||||
Lupo et al (2017) | √ | |||||
Notario-Pérez et al (2019) | √ | |||||
aNotario-Pérez et al (2017) | √ | |||||
bNotario-Pérez et al (2017) | √ | |||||
Nowak et al (2015) | √ | √ | ||||
Pacheco-Quito et al (2020) | √ | √ | ||||
Paczkowska et al (2020) | √ | √ | ||||
Palade et al (2013) | – | – | – | – | – | – |
Patel A. et al (2012) | √ | √ | ||||
Patel A. et al (2011) | √ | √ | ||||
Patel G.M. et al (2010) | √ | √ | ||||
Pendekal et al (2013) | √ | √ | ||||
Pendekal et al (2012) | √ | √ | ||||
Perioli et al (2009) | √ | √ | ||||
Perioli et al (2011) | √ | √ | ||||
Sánchez et al (2017) | √ | √ | ||||
Szymańska et al (2014) | √ | √ | ||||
Tunpanich et al (2019) | √ | |||||
Valenta et al (2001) | √ |
A simple apparatus called the dynamometer was used to perform the forced detachment test in several studies (30,33,50). Some research articles have devised a simple apparatus by modifying an analytical balance to measure the force required to remove the vaginal tablet from the vaginal tissue. An example of this apparatus setup is shown in Figure 8. Any modifications can be done according to what is available to the researchers. Therefore, only a general apparatus setup will be discussed. One arm of the balance is used to hold the vaginal tablet and contact the vaginal tissue fixed on a plank/platform. A pre-load force is used to ensure adhesion bond formation when contact is made. The other arm is used to place the weights that will be added until the tablet is pulled apart from the vaginal tissue by the weights’ gravity (26). In some cases, researchers used addition of water at a constant rate, instead of weights (12,38). The addition of water is stopped when the tablet detaches from the vaginal tissue. The weight required to detach the tablet formulation from the mucosa was noted (26).
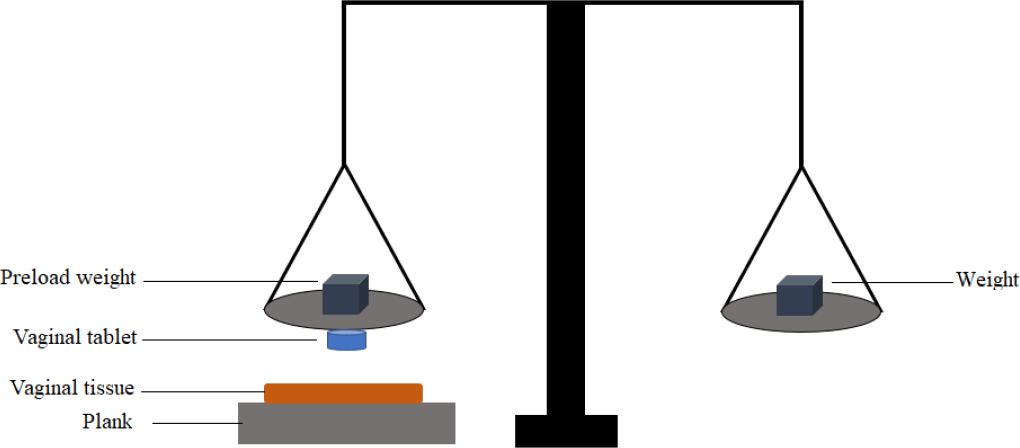
Fig. 8 - A schematic diagram of the modified analytical balance devise to measure the weight required to detach the mucoadhesive vaginal tablet from the vaginal tissue.
Time measurement test
An optimal vaginal tablet formulation must not only be able to have a good drug release control but must also remain adhered to the vaginal mucosa for a similar period of time as the drugs are released to be therapeutically effective (16). A total of 15 research articles in this review have conducted an in vitro/ex vivo test to measure how long the vaginal tablets remain attached to the vaginal mucosa. This assessment was conducted in a few different ways, by (i) total immersion, (ii) modified disintegration apparatus, and (iii) wash-off method. The mucoadhesion time was assessed over time by observation of the samples, including erosion and complete detachment of the tablet from the vaginal mucosa (16). Although there are a few different methods the general principle of a detachment test involves first attaching the vaginal tablet to the vaginal tissue, then immersing them in a dissolution solvent. This then is kept at body temperature of 37 ± 1°C in an incubator or a water bath with or without agitation depending on the study. Visually, the time taken for erosion and completed detachment of the vaginal tablet is recorded as the mucoadhesion retention time (34).
The method by total immersion involves mounting the vaginal tissue onto a glass slide or stainless steel plate using cyanoacrylate glue. A vaginal tablet was wetted and allowed to attach to the vaginal tissue with a slight force (14). The glass slide can then be inserted in a beaker containing the dissolution solvent, at an angle (Fig. 9) or vertically with an aid of a clamp. Figure 9 shows a schematic diagram of the total immersion method at an angle to measure the mucoadhesion time of a mucoadhesive vaginal tablet formulation. Illustration includes the swelling, formation of gel layer, and detachment of the tablet. (Picture was taken from Pacheco-Quito et al (11), Figure 8, page 12 of 19 and reproduced under the Creative Common Attribution License.)
A study by Hani et al (38) glued the vaginal tissue directly on the inner side of the beaker, attached a vaginal tablet on to the tissue, and filled the beaker with dissolution solvent. This assemble was then left in a shaking incubator (38).
In vivo mucoadhesive assessment
The in vivo mucoadhesive studies include administering the vaginal tablet intravaginally to live healthy animal models, typically rats or rabbits. It is primarily applied to evaluate the behavior of vaginal tablets (appearance, physical changes, swelling behavior, and residence time in vagina) (25). The physical status of the tablets can be observed at certain time intervals using various methods including vaginal speculum (25), pictures taken using laparoscopic probe with a camera (27), and x-ray (51), as shown in Figure 10. The images illustrate that the vaginal tablet was able to swell, remains intact, and adhered to the vaginal mucosa (51). (Pictures are taken from (51), Figure 8, page 184 and reproduced under the Creative Commons license.)

Fig. 9 - A schematic diagram showing the total immersion method at an angle to measure the mucoadhesion time of a mucoadhesive vaginal tablet formulation. Illustration includes the swelling, formation of a gel layer, and detachment of the tablet. (Picture was taken from Pacheco-Quito et al (11), Figure 8, page 12 and 19 and reproduced under the Creative Common Attribution License.)
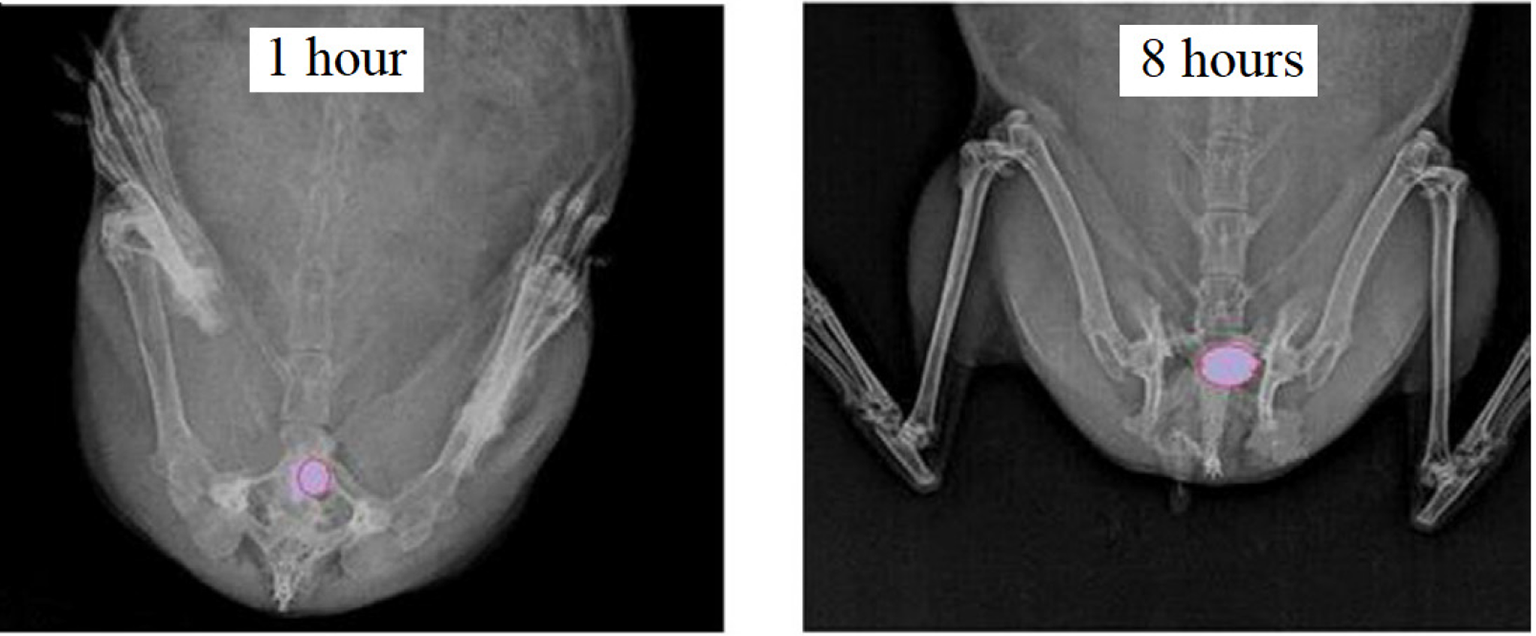
Fig. 10 - X-ray radiographic images of a rabbit’s vaginal cavity after 1 and 8 hours of vaginal tablet administration. The images demonstrate that this vaginal tablet formulation was able to swell, remains intact, and adhered to the vaginal mucosa over the time allowed (51). (Pictures are taken from (51), Figure 8, page 184 and reproduced under the Creative Commons license.)
From this assessment, the retention time of the tablet formulation can be established in vivo and evidently show that the tablet can remain intact and adhered to the vaginal mucosa for an expected amount of time (27). Some research articles expanded the in vivo assessment by pharmacokinetic evaluation of the drug substance present in the blood and plasma of the animal taken at different time intervals (12,14) and histological examinations (12). This can then be correlated with the drug release profile of the tablet formulation.
Simulated vaginal fluid
To optimize the formulations destined for the vaginal site, a reliable in vitro method must be put in place that may better mimic the real biological environment in the vagina, in particular in the presence of vaginal fluids. This can be essential as to better understand the behavior of the formulated tablets in the target site (47). The vaginal fluid itself has become an essential tool to evaluate the mucoadhesive strength of the formulation. It demonstrates the interaction between the vaginal fluid to the formulation. Furthermore, it helps to better understand the behavior of the formulated tablets at the target site. Therefore, simulated vaginal fluid (SVF) is used in many methods (e.g., swelling studies, dissolution studies). Vaginal fluid originates from a number of different sources. The fluid is mostly transudate from vaginal and cervical cells and also contains vulvar secretions from sebaceous, sweat, Bartholin, and skene glands, cervical mucus, endometrial and oviductal fluids, microorganisms, and their metabolic products (47). Because of the limited quantity of human vaginal fluid and its rapid degradation once collected from its source, researchers have developed a SVF in order to model the fluid properties originating in the vagina (47). Many research articles had referred and modified the SVF proposed by Owen and Katz’s original research (52). A liter of SVF is prepared using NaCl (3.51 g), KOH (1.40 g), Ca(OH)2 (0.222 g), albumin (0.18 g), acetic acid (1.00 g), lactic acid (2.0 g), glycerol (0.16 g), urea (0.4 g), glucose (5.0 g) mixed in 1000 mL water and stirred well until complete dissolution (14). pH of the SVF can be adjusted to 4–5 with either HCl or acetic acid according to different research articles.
Other relevant assessments
There are many other assessment methods that were conducted in the research articles reviewed, as reported in Table IX. We believe they contribute additional information to strengthen the sense of the formulated tablets in terms of stability and performance.
Physicochemical interaction studies
In this type of study, the compatibility of the drug substance and the excipients are assessed. Investigations can be performed using Fourier transform infrared spectrometry (FTIR) and/or differential scanning calorimetry (DSC) on drugs and excipients separately and in a mixed state. The results from these techniques will provide the degree of compatibility between the drug substance–excipient as well as excipient–excipient (38). It can be concluded that there are no chemical interactions in the mixes tested, if there are no changes in the characteristic peaks obtained in the IR spectra and DSC thermograms of individual substances (18,38).
Ex vivo permeation study
A research article by Pendekal et al (51) conducted an ex vivo permeation study that was carried out for the optimized formulation using Franz diffusion cell. The tablet was placed in the donor compartment on the sheep mucosa. The mucosal layer is on donor compartment. The receptor compartment was filled with a dissolution solvent and the temperature was maintained at 37 ± 0.5°C and 50 rpm. The amount of drug substances permeated through the sheep mucosa was determined by taking sample aliquots from the receptor compartment using a syringe and immediately replacing the same volume of solvent (51).
Author | DSC | FTIR | NMR | Size analysis | Rheology | SEM |
---|---|---|---|---|---|---|
Abidin et al (2020) | √ | √ | ||||
Abu El-Enin et al (2020) | – | – | – | – | – | – |
Baki et al (2009) | – | – | – | – | – | – |
Baloglu et al (2011) | √ | |||||
Bartkowiak et al (2018) | – | – | – | – | – | – |
Bhat et al (2010) | √ | √ | ||||
Cazorla-Luna et al (2019) | – | – | – | – | – | – |
Cevher et al (2014) | √ | √ | ||||
Cevher et al (2008) | √ | √ | √ | √ | ||
El-Kamel et al (2002) | – | – | – | – | – | – |
Fitaihi et al (2017) | – | – | – | – | – | – |
Gupta et al (2013) | √ | √ | √ | √ | ||
Gök et al (2017) | – | – | – | – | – | – |
Hani et al (2016) | √ | √ | ||||
Hassan et al (2017) | – | – | – | – | – | – |
Hombach et al (2009) | √ | √ | ||||
Kailasam et al (2010) | – | – | – | – | – | – |
Kast et al (2002) | – | – | – | – | – | – |
Khan et al (2017) | √ | |||||
Khan et al (2014) | √ | |||||
Lupo et al (2017) | √ | √ | ||||
Notario-Pérez et al (2019) | – | – | – | – | – | – |
aNotario-Pérez et al (2017) | – | – | – | – | – | – |
bNotario-Pérez et al (2017) | – | – | – | – | – | – |
Nowak et al (2015) | – | – | – | – | – | – |
Pacheco-Quito et al (2020) | √ | √ | ||||
Paczkowska et al (2020) | – | – | – | – | – | – |
Palade et al (2013) | – | – | – | – | – | – |
Patel A. et al (2012) | – | – | – | – | – | – |
Patel A. et al (2011) | – | – | – | – | – | – |
Patel G.M. et al (2010) | – | – | – | – | – | – |
Pendekal et al (2013) | √ | √ | ||||
Pendekal et al (2012) | √ | √ | ||||
Perioli et al (2009) | √ | |||||
Perioli et al (2011) | √ | |||||
Sánchez et al (2017) | – | – | – | – | – | – |
Szymańska et al (2014) | √ | √ | ||||
Tunpanich et al (2019) | √ | √ | ||||
Valenta et al (2001) | √ |
DSC = differential scanning calorimetry; FTIR = Fourier transform infrared spectrometry; NMR = nuclear magnetic resonance; SEM = scanning electron microscopy.
Limitations
The initial strategy was to exclude any unaccessible articles to reduce the limitation in conducting this review. There were no research articles that were unaccessible. The authors also acknowledge the large number of articles selected for this review. However, it was thought that it helps to map out the tests available in evaluating a mucoadhesive vaginal tablet formulation.
Conclusions
Although drug-controlled release profiles, mucoadhesion force, and mucoadhesion residence periods are utilized to determine the optimal formulation, vaginal formulations must be created for women’s convenience, which can enhance patient compliance. Because the system must have two unique properties: (i) immobilization and (ii) controlled release characteristics, mucoadhesive drug delivery is quite complex. As a result, the approaches discussed in this study can be used to assess the balance between the two features without sacrificing one. Understanding the goals and concepts of each assessment approach can aid researchers in evaluating experimental formulations and obtaining an optimal formulation more rapidly.
Disclosures
Funding: Funding for this work came from the AIT President’s Seed Fund (PSF2017-IM).
Conflict of interest: The authors declare no conflict of interest.
Author contributions: I.M. and E.J.M. provided relevant references and resources. I.Z.A. and G.W.F. compiled, interpreted, and analyzed the data. I.Z.A., E.J.M., and I.M. wrote the article. All authors revised the article and gave final approval of the version to be submitted. All authors have read and agreed to the published version of the manuscript.
References
- 1. das Neves J, Palmeira-de-Oliveira R, Palmeira-de-Oliveira A, Rodrigues F, Sarmento B. Vaginal mucosa and drug delivery. In: Khutoryanskiy VV, ed. Mucoadhesive materials and drug delivery systems. Chichester: John Wiley & Sons 2014; 99-132. CrossRef
- 2. das Neves J, Notario-Pérez F, Sarmento B. Women-specific routes of administration for drugs: a critical overview. Adv Drug Deliv Rev. 2021;176:113865. CrossRef PubMed
- 3. de Araújo Pereira RR, Bruschi ML. Vaginal mucoadhesive drug delivery systems. Drug Dev Ind Pharm. 2012;38(6):643-652. CrossRef PubMed
- 4. Acartürk F. Mucoadhesive vaginal drug delivery systems. Recent Pat Drug Deliv Formul. 2009;3(3):193-205. CrossRef PubMed
- 5. Bartkowiak A, Rojewska M, Hyla K, Zembrzuska J, Prochaska K. Surface and swelling properties of mucoadhesive blends and their ability to release fluconazole in a mucin environment. Colloids Surf B Biointerfaces. 2018;172:586-593. CrossRef PubMed
- 6. Vermani K, Garg S. The scope and potential of vaginal drug delivery. Pharm Sci Technol Today. 2000;3(10):359-364. CrossRef PubMed
- 7. Zhang L, De Salvo R, Ehret A, Young K, Trapp S. Vulvovaginal candidiasis: a real-world evidence study of the perceived benefits of Canesten®. SAGE Open Med. 2022;10:20503121221085437. CrossRef PubMed
- 8. McCoy CF, Spence P, Dallal Bashi YH, et al. Use of simulated vaginal and menstrual fluids to model in vivo discolouration of silicone elastomer vaginal rings. Int J Pharm X. 2021;3:100081. CrossRef PubMed
- 9. Chollet JA. Efficacy and safety of ultra-low-dose Vagifem (10 mcg). Patient Prefer Adherence. 2011;5:571-574. CrossRef PubMed
- 10. Machado RM, Palmeira-de-Oliveira A, Gaspar C, Martinez-de-Oliveira J, Palmeira-de-Oliveira R. Studies and methodologies on vaginal drug permeation. Adv Drug Deliv Rev. 2015;92:14-26. CrossRef PubMed
- 11. Pacheco-Quito E-M, Ruiz-Caro R, Rubio J, Tamayo A, Veiga MD. Carrageenan-based acyclovir mucoadhesive vaginal tablets for prevention of genital herpes. Mar Drugs. 2020;18(5):249. CrossRef PubMed
- 12. Hassan AS, Soliman GM, Ali MF, El-Mahdy MM, El-Gindy GEA. Mucoadhesive tablets for the vaginal delivery of progesterone: in vitro evaluation and pharmacokinetics/pharmacodynamics in female rabbits. Drug Dev Ind Pharm. 2018;44(2):224-232. CrossRef PubMed
- 13. Cevher E, Açma A, Sinani G, Aksu B, Zloh M, Mülazımoğlu L. Bioadhesive tablets containing cyclodextrin complex of itraconazole for the treatment of vaginal candidiasis. Int J Biol Macromol. 2014;69:124-136. CrossRef PubMed
- 14. Abu El-Enin ASM, Elbakry AM, El Hosary R, Fouad Lotfy MA, Yahia R. Formulation, development, in vivo pharmacokinetics and pharmacological efficacy evaluation of novel vaginal bioadhesive sustained core-in-cup salbutamol sulphate tablets for preterm labor. J Drug Deliv Sci Technol. 2020;60:102076. CrossRef
- 15. Garg S, Goldman D, Krumme M, Rohan LC, Smoot S, Friend DR. Advances in development, scale-up and manufacturing of microbicide gels, films, and tablets. Antiviral Res. 2010;88(suppl 1):S19-S29. CrossRef PubMed
- 16. Cazorla-Luna R, Notario-Pérez F, Martín-Illana A, et al. Chitosan-based mucoadhesive vaginal tablets for controlled release of the anti-HIV drug tenofovir. Pharmaceutics. 2019;11(1):20. CrossRef PubMed
- 17. Clark MR, Peet MM, Davis S, Doncel GF, Friend DR. Evaluation of rapidly disintegrating vaginal tablets of tenofovir, emtricitabine and their combination for HIV-1 prevention. Pharmaceutics. 2014;6(4):616-631. CrossRef PubMed
- 18. Abidin IZ, Rezoagli E, Simonassi-Paiva B, et al. A bilayer vaginal tablet for the localized delivery of disulfiram and 5-fluorouracil to the cervix. Pharmaceutics. 2020;12(12):1185. CrossRef PubMed
- 19. Higgins JP, Green S. Cochrane handbook for systematic reviews of interventions. Chichester: John Wiley & Sons; 2019. CrossRef
- 20. Lee C-H, Chien YW. Development and evaluation of a mucoadhesive drug delivery system for dual-controlled delivery of nonoxynol-9. J Control Release. 1996;39(1):93-103. CrossRef
- 21. Perrone M, Lopalco A, Lopedota A, et al. S-preactivated thiolated glycol chitosan useful to combine mucoadhesion and drug delivery. Eur J Pharm Biopharm. 2018;132:103-111. CrossRef PubMed
- 22. Rojewska M, Bartkowiak A, Milanowski B, Prochaska K, Lulek J. Physicochemical and release studies of new mucoadhesive fluconazole delivery systems. Colloids Surf A Physicochem Eng Asp. 2019;566:11-20. CrossRef
- 23. Szymańska E, Winnicka K, Amelian A, Cwalina U. Vaginal chitosan tablets with clotrimazole-design and evaluation of mucoadhesive properties using porcine vaginal mucosa, mucin and gelatine. Chem Pharm Bull (Tokyo). 2014;62(2):160-167. CrossRef PubMed
- 24. Cevher E, Sensoy D, Zloh M, Mülazimoğlu L. Preparation and characterisation of natamycin: γ-cyclodextrin inclusion complex and its evaluation in vaginal mucoadhesive formulations. J Pharm Sci. 2008;97(10):4319-4335. CrossRef PubMed
- 25. Gök MK, Demir K, Cevher E, et al. The effects of the thiolation with thioglycolic acid and l-cysteine on the mucoadhesion properties of the starch-graft-poly(acrylic acid). Carbohydr Polym. 2017;163:129-136. CrossRef PubMed
- 26. Bhat S, Shivakumar H. Bioadhesive controlled release clotrimazole vaginal tablets. Trop J Pharm Res. 2010;9:339-346. CrossRef
- 27. Gupta NV, Natasha S, Getyala A, Bhat RS. Bioadhesive vaginal tablets containing spray dried microspheres loaded with clotrimazole for treatment of vaginal candidiasis. Acta Pharm. 2013;63(3):359-372. CrossRef PubMed
- 28. Tunpanich P, Limpongsa E, Pongjanyakul T, Sripanidkulchai B, Jaipakdee N. Mucoadhesive sustained-release tablets for vaginal delivery of Curcuma comosa extracts: preparation and characterization. J Drug Deliv Sci Technol. 2019;51:559-568. CrossRef
- 29. Notario-Pérez F, Martín-Illana A, Cazorla-Luna R, et al. Influence of chitosan swelling behaviour on controlled release of tenofovir from mucoadhesive vaginal systems for prevention of sexual transmission of HIV. Mar Drugs. 2017;15(2):50. CrossRef PubMed
- 30. Perioli L, Ambrogi V, Pagano C, Scuota S, Rossi C. FG90 chitosan as a new polymer for metronidazole mucoadhesive tablets for vaginal administration. Int J Pharm. 2009;377(1-2):120-127. CrossRef PubMed
- 31. Kailasam P, Jamunadhevi V, Kaur G. Formulation and evaluation of once daily mucoadhesive vaginal tablet of metronidazole. Int J Res Pharm Sci. 2010;1:308-312. Online
- 32. Fitaihi RA, Aleanizy FS, Elsamaligy S, Mahmoud HA, Bayomi MA. Role of chitosan on controlling the characteristics and antifungal activity of bioadhesive fluconazole vaginal tablets. Saudi Pharm J. 2018;26(2):151-161. CrossRef PubMed
- 33. Sánchez MT, Ruiz MA, Castán H, Morales ME. A novel double-layer mucoadhesive tablet containing probiotic strain for vaginal administration: Design, development and technological evaluation. Eur J Pharm Sci. 2018;112:63-70. CrossRef PubMed
- 34. Khan AB, Thakur RS. Design and evaluation of mucoadhesive vaginal tablets of tenofovir disoproxil fumarate for pre-exposure prophylaxis of HIV. Drug Dev Ind Pharm. 2018;44(3):472-483. CrossRef PubMed
- 35. El-Kamel A, Sokar M, Naggar V, Al Gamal S. Chitosan and sodium alginate-based bioadhesive vaginal tablets. AAPS PharmSci. 2002;4(4):E44. CrossRef PubMed
- 36. Baloglu E, Ay Senyıgıt Z, Karavana SY, et al. In vitro evaluation of mucoadhesive vaginal tablets of antifungal drugs prepared with thiolated polymer and development of a new dissolution technique for vaginal formulations. Chem Pharm Bull (Tokyo). 2011;59(8):952-958. CrossRef PubMed
- 37. Paczkowska M, Chanaj-Kaczmarek J, Romaniuk-Drapała A, et al. Mucoadhesive chitosan delivery system with Chelidonii herba lyophilized extract as a promising strategy for vaginitis treatment. J Clin Med. 2020;9(4):1208. CrossRef PubMed
- 38. Hani U, Shivakumar HG, Osmani RA, Srivastava A, Kumar Varma NS. Development of a curcumin bioadhesive monolithic tablet for treatment of vaginal candidiasis. Iran J Pharm Res. 2016;15(1):23-34. PubMed
- 39. Shah RB, Tawakkul MA, Khan MA. Comparative evaluation of flow for pharmaceutical powders and granules. AAPS PharmSciTech. 2008;9(1):250-258. CrossRef PubMed
- 40. Jallo LJ, Ghoroi C, Gurumurthy L, Patel U, Davé RN. Improvement of flow and bulk density of pharmaceutical powders using surface modification. Int J Pharm. 2012;423(2):213-225. CrossRef PubMed
- 41. Beakawi Al-Hashemi HM, Baghabra Al-Amoudi OS. A review on the angle of repose of granular materials. Powder Technol. 2018;330:397-417. CrossRef
- 42. Cazorla-Luna R, Martín-Illana A, Notario-Pérez F, et al. Vaginal polyelectrolyte layer-by-layer films based on chitosan derivatives and Eudragit® S100 for pH responsive release of tenofovir. Mar Drugs. 2020;18(1):44. CrossRef PubMed
- 43. Notario-Pérez F, Cazorla-Luna R, Martín-Illana A, et al. Optimization of tenofovir release from mucoadhesive vaginal tablets by polymer combination to prevent sexual transmission of HIV. Carbohydr Polym. 2018;179:305-316. CrossRef PubMed
- 44. Jug M, Hafner A, Lovrić J, et al. An overview of in vitro dissolution/release methods for novel mucosal drug delivery systems. J Pharm Biomed Anal. 2018;147:350-366. CrossRef PubMed
- 45. Palade L, Popovici I, Cojocaru I. In vitro evaluation of 5-fluorouracil dissolution profiles from vaginal bioadhesive tablets. Farmacia. 2013;61(4):640-647.
- 46. Baki G, Bajdik J, Kelemen A, Pintye-Hódi K. Formulation of a solid intravaginal matrix system to prolong the pH-decreasing effect of lactic acid. J Drug Deliv Sci Technol. 2009;19(2):133-137. CrossRef
- 47. Fernandes L, Costa R, Henriques M, Rodrigues ME. Simulated vaginal fluid: candida resistant strains’ biofilm characterization and vapor phase of essential oil effect. J Mycol Med. 2022;33(1):101329. CrossRef PubMed
- 48. Lupo N, Fodor B, Muhammad I, Yaqoob M, Matuszczak B, Bernkop-Schnürch A. Entirely S-protected chitosan: a promising mucoadhesive excipient for metronidazole vaginal tablets. Acta Biomater. 2017;64:106-115. CrossRef PubMed
- 49. Colombo N, Carinelli S, Colombo A, et al. ESMO Guidelines Working Group. Cervical cancer: ESMO Clinical Practice Guidelines for diagnosis, treatment and follow-up. Ann Oncol. 2012; Suppl 7:vii27-32. CrossRef PubMed
- 50. Perioli L, Ambrogi V, Pagano C, Massetti E, Rossi C. New solid mucoadhesive systems for benzydamine vaginal administration. Colloids Surf B Biointerfaces. 2011;84(2):413-420. CrossRef PubMed
- 51. Pendekal MS, Tegginamat PK. Hybrid drug delivery system for oropharyngeal, cervical and colorectal cancer—in vitro and in vivo evaluation. Saudi Pharm J. 2013;21(2):177-186. CrossRef PubMed
- 52. Owen DH, Katz DF. A vaginal fluid simulant. Contraception. 1999;59(2):91-95. CrossRef PubMed