![]() |
Drug Target Insights 2022; 16: 25-35 ISSN 1177-3928 | DOI: 10.33393/dti.2022.2476 REVIEW |
Focus on Antimicrobial Resistance (AMR)
|
Understanding the environmental drivers of clinical azole resistance in Aspergillus species
ABSTRACT
Aspergilli are ubiquitous fungal pathogens associated with severe life-threatening infections, especially in immunocompromised patients. Azoles are the first line of defence in the fight against most Aspergillus-related infections. However, resistance to these therapeutic compounds has developed, which is mainly due to the existence of mutations in lanosterol 14 alpha-demethylase (Cyp51A), a crucial enzyme in the pathway that produces ergosterol and is the target of azole antifungals. Azole-based antifungal medications are ineffective because of infections brought on by azole-resistant Aspergillus species, leading to a high fatality rate. However, resistant Aspergillus isolates have also been isolated from azole-naïve patients. Global agricultural practices promote the use of azole fungicides to protect crops from phytopathogens. Usage of azole fungicides on a large scale has been linked to the development of resistance among Aspergillus species prevalent in the environment. The infections caused by these azole-resistant Aspergillus species cannot be treated by the available azole drugs, in turn leading to high morbidity and mortality rates. Thus, knowledge of the environmental drivers and comprehending the genetic basis of fungal drug resistance evolution is pertinent, considering increasing numbers of patients with COVID-19 infections who are sensitive to opportunistic fungal infections. This article emphasises the prevalence and underlying mechanisms of azole resistance in Aspergillus species, with a focus on environmental triggers and resistance development. It also highlights the need for regular surveillance of pesticide use in agriculture, detection of triazole-resistant Aspergillus species in environmental and clinical settings and development of new antifungal drugs.
Keywords: Aspergillus, Azole resistance, Biofilm, cyp51A gene, Environmental origin, Triazoles
Received: July 27, 2022
Accepted: October 24, 2022
Published online: November 22, 2022
Drug Target Insights - ISSN 1177-3928 - www.aboutscience.eu/dti
© 2022 The Authors. This article is published by AboutScience and licensed under Creative Commons Attribution-NonCommercial 4.0 International (CC BY-NC 4.0).
Commercial use is not permitted and is subject to Publisher’s permissions. Full information is available at www.aboutscience.eu
Introduction
The saprophytic genus Aspergillus is universal in the environment that releases large numbers of conidiospores. After inhalation, they either reach the terminal airways or settle in large groups in the upper ventilatory system and cause sensitisation. The small size of the spores, thermotolerance, resistance to oxidative damage and the capacity to create proteolytic or even immunosuppressive enzymes are among Aspergillus’ biological characteristics which permits growth at body temperature (1,2). Aspergillus species reported as human pathogens are Aspergillus fumigatus, Aspergillus niger, Aspergillus flavus and Aspergillus nidulans. Bronchitis, invasive aspergillosis (IA), and chronic pulmonary aspergillosis (CPA) are all signs of Aspergillus infection. In addition, severe asthma with fungal hypersensitivity and allergic bronchopulmonary aspergillosis (ABPA) are allergic symptoms of inhaled Aspergillus (3).
Current antifungal therapy for aspergillosis falls into three main categories: polyenes, echinocandins, and azoles. Of these, azoles are the drug of choice for treating Aspergillus infection. In addition, they are the only orally available antifungal agents for therapy and are essential for long-term treatment (3). A. fumigatus has developed azole resistance over the past few decades because of long-term azole therapy for aspergillosis in the clinical environment (4). Furthermore, azole-resistant isolates of Aspergillus detected in azole-naïve (5) individuals indicate that there may be a second route for the establishment of resistance through A. fumigatus azole fungicide exposure in agro ecosystems.
Global prevalence of Aspergillosis
Aspergillosis can be either chronic or acute. Most commonly, aspergillosis diseases are CPA, IA and bronchitis (6). Aspergillus species are responsible for >2,00,000 cases of IA annually, as shown in Table I (7).
Fungal disease | Global burden | Comments |
---|---|---|
Asthmatic allergic bronchopulmonary aspergillosis | ~4,800,000 | Adults only, rare in children |
Cystic fibrosis–related allergic bronchopulmonary aspergillosis | ~6675 | Adults only, starts from age 4 |
Invasive aspergillosis | ~3,00,000 | About 10 million at risk annually |
Chronic pulmonary aspergillosis | ~3,000,000 |
In immunocompromised individuals, such as those with severe neutropenia, individuals who had bone marrow transplant or solid organ transplants, those with advanced acquired immunodeficiency syndrome or with chronic granulomatous illness, IA can develop (10). Invasion of the lungs by Aspergillus leads to tissue damage leading to sepsis and sometimes haemoptysis in later stages (11). In addition to these classic risk factors for IA, liver cirrhosis, tuberculosis, diabetes mellitus and persistent lung disease can also develop (12-15). CPA is an infectious disease that progressively damages lung tissue. This is especially true for immunocompromised people with a previous or underlying lung disease, such as tuberculosis or chronic obstructive pulmonary disease (COPD). Awareness of this debilitating and ultimately deadly infection is growing. It is tentatively estimated that there are 3 million CPA (Chronic pulmonary aspergillosis) patients worldwide (6). Among Asian countries, the highest CPA burden was recorded in India (209,147) (15), followed by Pakistan (55,509) (16), Bangladesh (20,720) (17), Nepal (6,611) (18) and Sri Lanka (2,886) (19). Patients with ABPA frequently experience uncontrolled asthma or repeated infections brought on by bronchiectasis, which progresses to lung damage, respiratory failure, and ultimately death. ABPA contamination in the Indian subcontinent is summarised in Table II (9,21).
Antifungal agents to treat aspergillosis
In general, there are three different types of antifungal medications used to treat Aspergillus-related illnesses: polyenes (amphotericin B), azoles (itraconazole, voriconazole and posaconazole) and echinocandins (caspofungin).
Polyenes
Polyenes are large macrolide structures with amphipathic nature (Fig. 1). The oldest class of antifungal drugs are the polyenes, which include nystatin, amphotericin B and pimaricin. Of these, amphotericin B is the only drug used to treat systemic infections. Polyenes promote channelling in fungal membranes by interacting with sterols in cell membranes (ergosterol in fungal cells), which leads to changing the permeability of the membrane leading to the leaking of intracellular components. The major antifungal medication used to treat severe Aspergillus infections is amphotericin B. However, it has detrimental side effects including fever, chills, hypotension, tachypnoea, as well as renal toxicity such as renal ischaemia, hypokalaemia, tubular acidosis and reduced erythropoiesis in the kidney (10).
Country | Chronic pulmonary aspergillosis | Invasive aspergillosis | Allergic bronchopulmonary aspergillosis | |||
---|---|---|---|---|---|---|
Burden | Rate/100,000 | Burden | Rate/100,000 | Burden | Rate/100,000 | |
India (15) | 209,147 | 24 | – | – | 1,380,000 | 114 |
Pakistan (16) | 55,509 | 70 | 10,949 | 5.9 | 94,358 | 51 |
Bangladesh (17) | 20,720 | 41 | 5166 | 5.1 | 90,262 | 56 |
Nepal (18) | 6,611 | 24.2 | 1119 | 4.0 | 9546 | 35 |
Sri Lanka (19) | 2,886 | 14.4 | 229 | 1.1 | 10,344 | 49 |
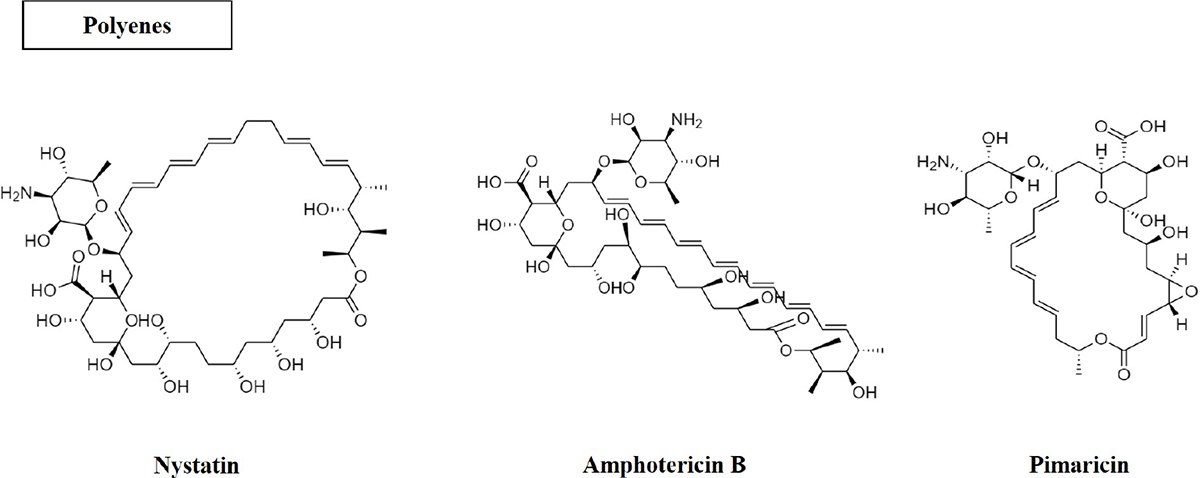
Fig. 1 - Structure of polyene antifungal compounds.
Azoles
The primary treatment includes azoles used to treat aspergillosis (20). This class of antifungals includes medicines with an azole ring (Fig. 2) that stops a variety of fungi from growing (22). Clotrimazole, econazole, ketoconazole, miconazole, and tioconazole are examples of two-nitrogen-atom imidazole. Triazoles, in contrast, have three nitrogen atoms in the azole ring (fluconazole, itraconazole, posaconazole and voriconazole). The antifungal effects of azole compounds were first described in 1944 (23). The first compound was imidazole, followed by triazole. The first azole antifungal that could be applied topically in a therapeutic setting was chlormidazole in 1958. Other imidazole-azole antifungals such as clotrimazole and miconazole were thereafter made available for topical application, and econazole was released in 1974 (24). The first drug was ketoconazole, an oral medication for systemic fungal infections, albeit its usage was constrained by its toxicity (25,26). Fluconazole and itraconazole, the two most used systemic triazoles, were first made available in the United States in 1990. Fluconazole and itraconazole have sufficient antifungal activity and are far less harmful than ketoconazole (27). Itraconazole and fluconazole are less toxic than ketoconazole and have adequate antifungal action (27). Voriconazole and posaconazole were the second-generation triazoles to be made available in the late 1990s and early 2000s. They have proven to be incredibly powerful against A. fumigatus (28) and are being utilised to manage fluconazole-resistant strains (29). Triazoles under investigation include albaconazole (30), isavuconazole and pramiconazole (31).
Azoles work by blocking the cytochrome P-450-dependent enzyme lanosterol demethylase, also known as 14α-sterol demethylase or P-450DM, which is necessary for the formation of ergosterol, a crucial component of fungal plasma membranes (32). Exposure to azoles in A. fumigatus decreases ergosterol levels and accumulates 14α-methylated sterols (33). This leads to a change in the structure and shape of the membrane, affects nutrient uptake and chitin synthesis, and inhibits fungal growth (34,35). On fungal cells, ergosterol also exhibits hormone-like characteristics that promote growth and reproduction (34). This function can also be impaired if the breakdown of ergosterol is more than 99% complete (34). Triazole is indispensable for long-term treatment, because of the fact that it is the only anti-Aspergillus drug that can be taken orally (36). Although itraconazole is still frequently used to treat the long-established non-invasive allergic type of aspergillosis (38), voriconazole is still advised as the first-line treatment for AI (34,35,37). Amphotericin B, a more lethal medication than triazoles, is the only available alternative.
Echinocandins
The most recent addition to the family of antifungal drugs are the echinocandins, with the first example, caspofungin, entering clinical use a decade ago (39). They are lipopeptide compounds that inhibit the enzyme (1,3) beta-D-glucan synthase, which produces glucan, the primary building block of fungal cell walls. Caspofungin (Fig. 3) is non-competitively effective against A. fumigatus, A. flavus and A. terreus. It is indicated for Aspergillus infections among those patients unresponsive or intolerant to other treatments. However, it has not been considered for first-line treatment (39).
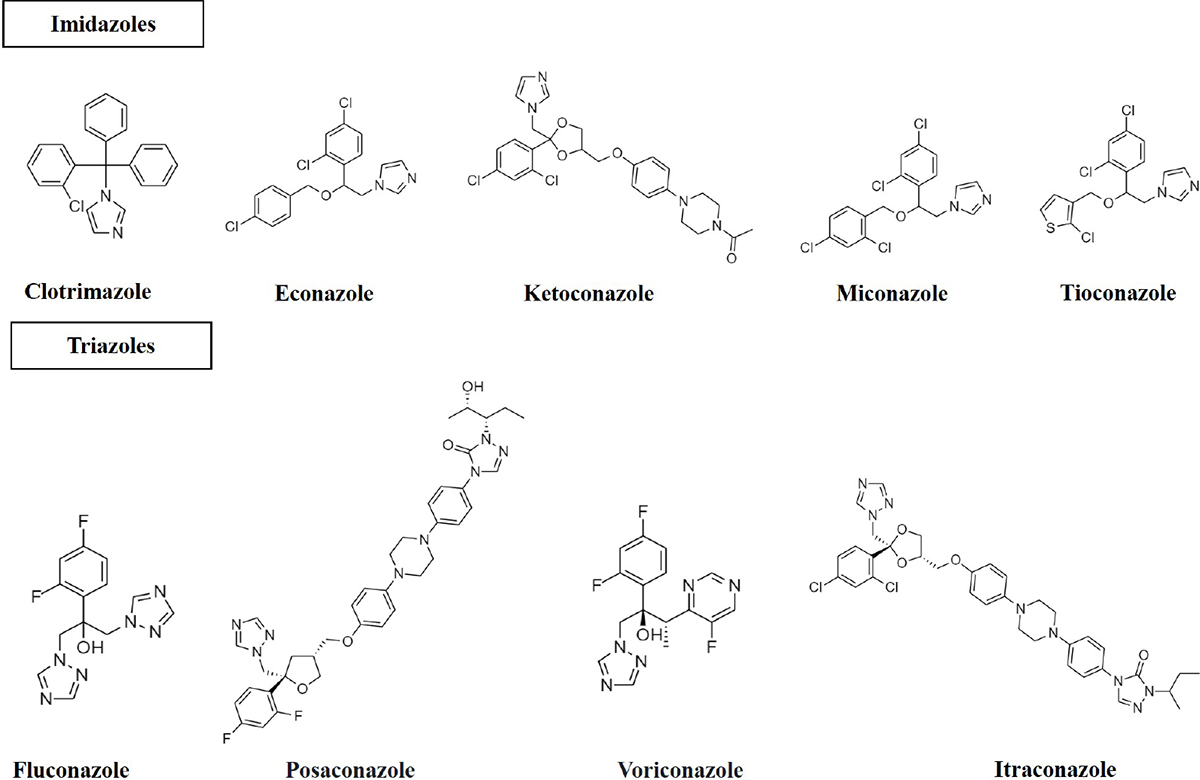
Fig. 2 - Structure of azole antifungals (imidazoles; two nitrogen in the azole ring and triazoles; three nitrogen in the azole ring).
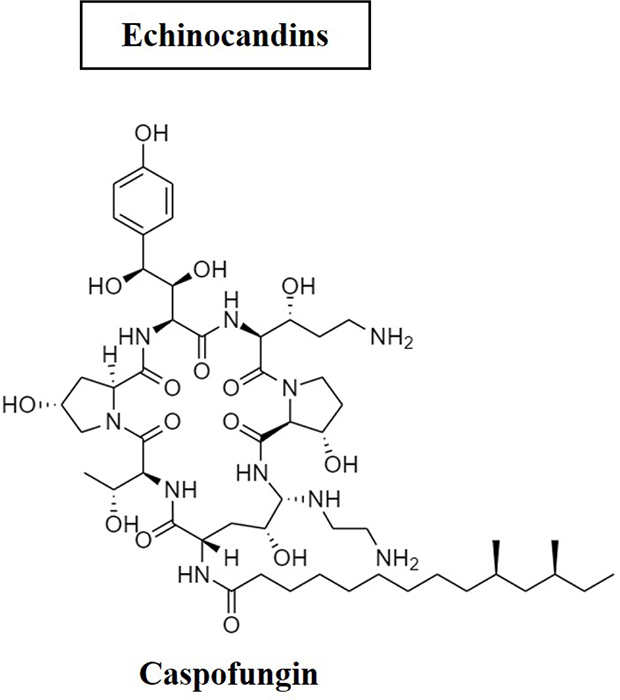
Fig. 3 - Structure of caspofungin antifungal.
Triazoles in the agricultural ecosystem
Plants are attacked by various pathogenic fungi that cause a variety of disease such as late blight, powdery mildew, leaf spot, blast, downy mildew, fruit rot, rust, etc. Throughout the world, the usage of synthetic insecticides and pesticides is quite common to overcome crop diseases and failure. However, these can have a significant negative impact on the environment (40). Thousands of tonnes of azoles are sold every year for crop protection. The advantages of azole pesticides include low cost and a diverse range of antifungal activity (41). Hexaconazole, propiconazole, triadimefon and tricyclazole are some of the prominent triazole fungicides available in the international market (42). The long-term stability of azoles is a crucial characteristic. With very slight modifications to their chemical composition, several azoles can continue to function for months in agricultural habitats (soil and water). Singh and Dureja (43) reported that hexaconazole can remain in soil for a long time due to its hydrophobic properties. Azoles are effective against several plant fungal diseases listed in Table II. The major metabolite of triadimefon, triadimenol, has a half-life in soil that varies from 110 to 375 days. The plants are sprayed several times per growing season at a dose of 100 g/ha, which is recommended to combat fungal diseases (41). There are currently 32 commercially available azole fungicides for crop protection (44). Several foods have been found to contain azole residues, for example, in samples of commercially available strawberries, grapes or mint. Therefore, there is evidence that large amounts of antifungal residues, especially azoles, can remain in the environment.
Azole fungicides | Crops | Diseases caused by Aspergillus |
---|---|---|
Triadimefon | Wheat, pea, grapes, coffee, mango, chilies, soybean | Bunt of wheat, powdery mildew, rust, powdery mildew, coffee rust, rust |
Bitertanol | Apple, groundnut, tea, wheat, groundnut | Scab, rust, tikka, blister blight, Karnal bunt |
Flusilazole | Grapes, apple, rice, chilies | Powdery mildew, scab, sheath blight |
Hexaconazole | Apple, rice, groundnut, mango, soybean, tea | Blister blight, powdery mildew rust, scab, blast, sheath blight, tikka leaf spot |
Tebuconazole | Wheat, groundnut, chili, rice | Blast sheath blight, loose smut, flag smut, collar rot, root rot, stem rot, fruit rot, powdery mildew |
Difenoconazole | Apple, groundnut, rice, chili, cumin, onion | Fruit rot, blight powdery mildew, sheath blight, scab, leaf spot rust, purple blotch |
Tricyclazole | Paddy | Blast |
Resistance to azoles developing in Aspergillus clinical isolates
Recent years have seen a rise in the concern of azole therapy resistance in patients with Aspergillus infections. A. fumigatus, which causes around 80% of invasive infections, has been found to possess the highest azole resistance (45); Azole resistance has also been demonstrated in other species including A. niger, A. terreus and A. flavus (46). Infections with resistant Aspergillus strains lead to the effectiveness of azole antifungals, resulting in high mortality rates. Azole-resistant strains of Aspergillus were reported in the United States in the late 1990s (47). There have been numerous reports of infections with resistant strains in Europe, particularly in the UK and the Netherlands. Since that time, practically every European nation has reported cases of azole resistance, including Germany, Ireland, Italy, Austria, Denmark, France, Sweden, Portugal, Spain and Turkey (25,48-64). A surveillance study from the UK reported that the prevalence of azole resistance in A. fumigatus increased from 0.43% in 1998-2011 to 2.2% in 2015-2017 (65). A multicentre study conducted in Taiwan found a 4% prevalence rate for A. fumigatus resistant to azoles (66). Among Asian countries, Taiwan and China were the first to report resistance to azoles (67,68). Furthermore, this study has sparked significant worries about the use of azole antifungal medications to treat IA in the future.
The increase in resistance to triazoles in the clinical setting can be explained by two main phenomena: (a) an azole-resistant Aspergillus strain was found after long-term treatment with azoles in patients with cavernous lung disease and aspergilloma. When susceptible Aspergillus strains acquire resistance to the pharmacological stress response of prolonged azole therapy, they develop resistance. Numerous point mutations were found in the azole-resistant A. fumigatus isolate, especially at codons 54 and 220 of cyp51A (69). Several resistance mechanisms associated with cyp51A have been identified in patients specifically associated with azole therapy (70). Human-to-human transmission is therefore highly unlikely and spread of resistance is very rare.
(b) Azole fungicides are frequently employed to protect crops in agricultural settings and are structurally like medicinal triazoles. Aspergillus species are found in soil together with other plant pathogens. Azoles that attack plant pathogens can also affect Aspergillus species found in the same ecosystem (3). Fungicides used repeatedly over a long period of time can create persistent selection pressure and lead to the development of resistant Aspergillus species. As a result, the environment contains Aspergillus species that are azole-resistant. When these conidia are inhaled by susceptible individuals, Aspergillus species become resistant to triazoles used for treatment. Several cases of triazole-resistant aspergillosis in humans and animals without prior triazole treatment have been reported worldwide (71,72).
Resistance to triazoles in Aspergillus clinical isolates is associated with the use of azole fungicides in agriculture
Many major agricultural fungi have developed resistance because of the site-specific mode of action and widespread application of 14α-demethylase inhibitor (DMI) fungicides to prevent post-harvest spoiling by plant-pathogenic fungus. Azole overuse in agriculture may have an impact on saprophytic microbiota species as well as plant-pathogenic fungi (73). The soil provides a natural habitat for several fungi that could be harmful, including Aspergillus, Coccidioides, Histoplasma and Cryptococcus. Recently, the use of azole pesticides has been identified as a significant contributing cause in the increasing prevalence of A. fumigatus isolates with a particular mechanism of resistance comprising the TR34/L98H mutation in the cyp51A gene.
Snelders et al.’s (74) findings that were obtained from both clinical and environmental sources exhibited cross-resistance to five triazole-DMI fungicides, notably bromuconazole, propiconazole, epoxiconazole, tebuconazole and difenoconazole, support the notion that Aspergillus species become resistant to triazoles as a result of environmental use of azole fungicide (74). Additionally, these researchers noted that all these five DMI-triazoles have efficacy against wild-type A. fumigatus but not against the resistant TR34/L98H A. fumigatus because of their molecular structure, which is similar to drug triazoles and when attached to the target enzyme, acquire a same conformation (74). In a related study from India, four of the five triazole DMIs – bromuconazole, tebuconazole, epoxiconazole and difenoconazole – showed substantially higher MIC (Minimum inhibitory concentration) values with TR34/L98H-resistant A. fumigatus from environmental and clinical samples than with wild-type non-resistant isolates. These drugs are known to have performance comparable molecular structures to drug triazoles (75).
In 2020, a study from India found that patients who had never taken triazole therapy had developed resistance, raising the possibility that environmental transmission may contribute to the emergence of resistance (5). Most of the resistance mechanisms found in patients without prior treatment with azole are resistance mechanisms associated with TR (tandem repeats). Most Aspergillus isolates from the environment also possessed this resistance mechanism (TR34/L98H or TR46/Y121F/T289A). Norway, the Netherlands, Denmark, the United Kingdom and India are some of the countries where all these resistant environmental isolates have been found (76). Thus, retrieval of a similar resistance mechanism from environmental isolates as patient isolates supported an environmental pathway for resistance development.
Environmental-induced mutations in azole-resistant Aspergillus isolates
Ergosterol is a crucial and distinct element of the fungal plasma membrane that gives the cell membrane stability and permeability. The enzyme cytochrome P450, also called sterol-14α-demethylase, converts lanosterol into ergosterol. cyp51A is a gene that codes for the cytochrome P450 enzyme. The ergosterol biosynthetic pathway is the general target of azole antifungals. Triazoles hinder the cytochrome P450 enzyme from performing its lanosterol-converting role in the ergosterol biosynthetic pathway, and causes the depletion of ergosterol and the deleterious build-up of lanosterol (74). Azole resistance is brought on by mutations in the cyp51A gene that change the cyp51A protein’s structure and reduce the enzymes’ affinity for azole therapies.
Various changes in cyp51A resulting in a pan-azole-resistant phenotype have been reported in A. fumigatus isolates from environment and clinical sources worldwide. The most frequent mechanisms of resistance reported in environmental and clinical strains of A. fumigatus are mutations in the TR34/L98H and TR46/Y121F/T289A genes. These changes in the cyp51A gene comprise TRs within the gene’s promoter region (77). The cyp51A gene is overexpressed because of the insertion of 34 base pair (bp) TRs (TR34) into the cyp51A gene’s promoter region and the substitution of the leucine 98 amino acid coding for histidine (TR34/L98H) (78). According to Table IV (77), TR34/L98H is the most typical resistance mechanism found in environmental and clinical strains of A. fumigatus in many different nations. Another resistance mechanism which has been proven is TR46/Y121F/T289A (Fig. 4) where a 46 bp TR promoter region of the cyp51A gene has substitutions of threonine 289 for alanine and tyrosine 121 for phenylalanine (TR46/Y121F/T289A), resulting in an elevated resistance to voriconazole in A. fumigatus (79). One of the most frequently detected mechanisms of resistance in environmental isolates from Europe are TR34/L98H and TR46/Y121F/T289A, and their emergence has already been connected to the widespread use of azole-based agricultural fungicides (tebuconazole, hexaconazole and epoxiconazole). Fungicide use is rising in India, where it already accounts for 19% of all pesticide use (80). Even though Europe is the worldwide leader in agricultural fungicide use (40%), it is followed by Japan and Latin America. In contrast to Europe, the United States uses very less azoles in agriculture (http://ec.europa.eu/food/fs/se/ssc/out278_en.pdf). Therefore, no TR34/L98H mutation has been reported in environmental clinical isolates in the United States, but this mutation has been found in the European setting and now also in India (77).
An environmental study conducted examined a variety of soil and air samples from different regions of India. In their study, soil samples were taken from natural soils (where no azole fungicides were used). The samples tested positive for Aspergillus strains, but no resistant isolates were detected. Their findings were supported with those of the Dutch environmental study, which discovered that none of the A. fumigatus isolates cultured in natural soil exhibited azole resistance (81). Therefore, commercialised compost and samples taken from fields where fungicides are consistently treated can be the focus of environmental research to find TR34/L98H mutations in A. fumigatus isolates.
In environmental samples from China (82), certain novel mutations (G448S, TR46/Y121F/T289A) with 46 bp triple TRs in the promoter region have been discovered. Other Asian nations like India, Iran and Kuwait have demonstrated azole resistance in environmental Aspergillus strains (83,84). About 2% of Aspergillus species in Kuwait were found to be azole resistant, according to another study on environmental resistance (84). Indian researchers have reported that environmental studies on azole resistance describe the TR46/Y121F/T289A mechanism (85), and the findings indicated that 44 out of 630 A. fumigatus isolates from soil of indoor air, paddy fields, tea gardens, cotton groves, flowerpots and hospitals were resistant and managed to retain TR34/L98H resistance (75). An environmental mechanism of resistance (TR46/Y121F/1289A) in strains of A. fumigatus was also first reported in this study. In 2009, a similar mutation was discovered in a Dutch patient and has also been reported in several patients from the Netherlands (86). This survey, which took place between 2012 and 2013, studied 105 environmental samples taken from North Indian agricultural fields. The study concluded that azole fungicide-treated agricultural soils in northern India co-occurred with TR34/L98H and TR46/Y121F/T289A. The identified Indian Aspergillus strains were likely to be highly adaptable recombinant descendants of a cross between a native azole-sensitive strain from within India and azole-resistant strain that migrated from outside India, followed by a mutation, according to genomic analysis of the Indian resistance mechanism TR34/L98H (75). Reports indicated a rapid spread of this mutation in Asia (83).
Geographic region/references | Sample | cyp51A resistance mechanisms |
---|---|---|
The Netherlands (81) | Soil | Unknown, F46Y/M172V/E427K |
France (87) | Dust from patients’ home | H285Y |
Germany (88) | Soil | G54A, M220I |
India (89) | Soil | G54E |
Taiwan (90) | Soil, air | Wild-type cyp51A or SNPs |
France (91) | Soil | Unknown, P216L |
Colombia (92) | Soil | TR46/Y121F/T289A, TR34/L98H and TR53 |
India (77) | Environment | TR34/L98H |
India (93) | Azole-naïve patient | G54E |
India (5) | Azole-naïve patient | G54R, P216L and Y431C |
SNP = single nucleotide polymorphism.
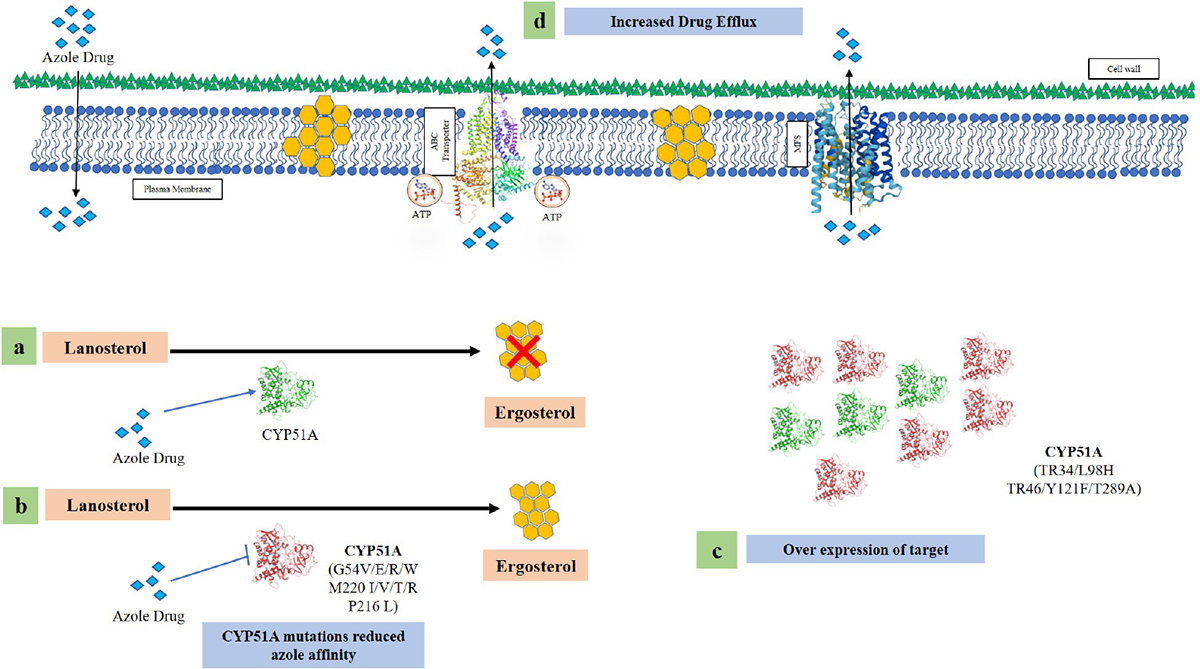
Fig. 4 - Various azole resistance mechanisms in Aspergillus fumigatus (a) Wild-type fungi in the presence of azole drug unable to make ergosterol. (b) Mutations in the cyp51A region alter the structural modifications of the enzyme leading to reduce azole affinity. (c) Insertion of 34 and 46 base pair in the promoter region along with point mutation in the cyp51A region causes overexpression of the gene. (d) Overexpression of efflux pump genes causing a reduced intracellular accumulation of azole drug.
Multiple triazole-resistant A. fumigatus isolates with the TR/L98H genotype were found in patients with chronic respiratory disease, according to a different study that was carried out for the first time in India (75). Only Europe and China have been described as having the TR/L98H mutation linked to pan-azole resistance in A. fumigatus (70,81). The two triazole-resistant A. fumigatus isolates are epidemiologically unrelated, share the same TR genotypes, and come from patients with no prior history of exposure to azoles or travel to Europe, suggesting that they most likely mutated and developed resistance as a result of exposure to the environment in India. The two isolates were phylogenetically distinct from TR/L98H, which contained the 25 A. fumigatus isolates from Dutch. The usage of azole fungicides in the environment may be a contributing factor in the propagation of this resistance mechanism (TR/L98H) in A. fumigatus isolates. A total of 43.7% of Aspergillus isolates found in 25 agricultural soil samples were found to be resistant to azoles, according to a recent environmental study in India (94).
Other non-synonymous hotspot mutations in the cyp51A gene have also been discovered in azole-resistant A. fumigatus strains, in addition to the TR34/L98H and TR46/Y121F/T289A alterations. While resistance to ITC (Itraconazole) and POS (Posaconazole) was provided by the glycine modification mutations 54 (G54) and 138 (G138), lower susceptibility to ITC and POS related to the glycine 448 (G448S) (95) mutation-related resistance to VRC. Methionine 220 (M220) amino acid substitution was also linked to a pronounced pattern of decreased sensitivity to triazoles (70). There have also been sporadic reports of other point mutations, including P216L, F219C, F219I, A284T, Y431C, G432S and G434C (86). Patients who received around 4 months (range 3 weeks to 23 months) of long-term azole treatment for persistent aspergillosis have been discovered to have the point mutations G54E/R/V and M220I/V/T/K (96). It is important to mention here that studies conducted in India, Tanzania, Romania and Germany found G54 mutations in environmental isolates of A. fumigatus (97). An environmental study in India found an azole-resistant Aspergillus species with a G54E mutation (89). This point mutation in the cyp51A gene is commonly seen in patients undergoing long-term azole therapy (89). In another study in India, A. fumigatus isolates with G54R, P216L and Y431C mutations were obtained from azole corpus patients (4). The MICs of many additional point mutations, including F46Y, M172V, N248T, D255E and E427K, have been discovered in azole-susceptible and azole-resistant Aspergillus isolates. However, this is not always restricted to clinical breaking point (75). The non-cyp51A pathway has also been linked to azole resistance in A. fumigatus. In isolates of A. fumigatus (98), voriconazole was also used to treat the link between biofilm growth and efflux pump activity to regulate homeostasis in azole resistance. Additionally, Aspergillus species can effectively invade and colonise the host by activating efflux pumps, specifically adenosine triphosphate (ATP)-binding cassette transporters and carriers of the major facilitator superfamily, to overcome the build-up of intercellular toxins (78).
Spread of Aspergillus from the environment to hospitals
Aspergillus spp. is ubiquitous in the environment and cosmopolitan in nature. The main habitat of Aspergillus spp. is the soil, and this saprophytic fungus has a vegetative mycelial life that develops on the decomposing matter, whether organic or vegetable, found in the soil (99). Previous studies showed that the metabolic machinery in Aspergillus spp. contains certain enzymes such as endo-β-glucanase, acetylxylan esterase, polygalacturonase, tannase, etc., which can easily degrade components of the plant cell wall. On the other hand, it does not contain any enzymes that can decompose plant wood (100). Spread of conidia occurs by asexual sporulation, and there is airborne spread of asexual reproductive organs, or conidia. Conidia mainly predominate in the air and are inhaled by individuals. It is estimated that 200 conidia are inhaled per person. However, they are stripped of pulmonary macrophages and neutrophils present in the lungs of immunocompetent humans. The clinical manifestations of Aspergillus depend on the host’s immune status. They cause severe infections in immunocompromised patients with other predisposing factors and develop life-threatening aspergillosis (101). A case study examined fatal IA and found that the source of Aspergillus infection was the patient’s home, which was in an agricultural area with potentially high pressure of fungicides used to protect crops. Even after the patient died, household samples showed the persistence of azole-resistant strains of Aspergillus spp. (63). Another study tested the source of azole-resistant Aspergillus spp. in a hospital environment. Their samples were taken from different environments in the hospital. The assessment showed the main source of Aspergillus spp. in the hospital and in the corridors (102), where the floor was decorated with tulip pots. This indicates the easy transmission of environmental Aspergillus strains to hospitals and infecting patients who were mainly in immunocompromised states or in persistent drug states. Therefore, it is important to identify sources of infection, whether the patient is hospitalised or a source of in-hospital contamination, due to the potential for aerosol transmission from patient to patient (103).
Azole-resistant Aspergillus biofilms
Aspergillus is an opportunistic airborne pathogen capable of forming biofilms in clinical settings or in immunocompromised patients with underlying conditions leading to allergic aspergillosis or IA (104). Biofilms are a community of cells strongly adherent to abiotic and biotic surfaces and surrounded by an extracellular matrix (ECM) composed of polysaccharides. The ECM acts as a protective sheet and external scaffold for adhesion and integration with the surface, and cell spreading for subsequent invasion. This protective layer becomes more sensitive to antifungal drug treatments and attacks immune cells, making them harder to fight (105). Possible factors contributing to drug resistance in Aspergillus spp. biofilms are: upregulation of efflux pump genes such as AfuMDR4, MDR1, MDR2, MDR4; induction of the HSP90 stress response pathway, which increases resistance to the antifungal drugs amphotericin B and caspofungin; by extracellular DNA which reduces drug sensitivity by preventing the drug from reaching its cellular target through ECM and sister cell formation while acting as drug-tolerant cells to form new biofilms (106).
The most important factor in IPA (Invasive pulmonary aspergillosis) and aspergilloma is biofilm formation. Fungal components such as drug transporters, secondary metabolites and cell wall components promote biofilm formation in host cells and are resistant to antifungal drug treatment (107). Biofilm formation, which helps to penetrate the host immune system and reduce the patient’s immune competence, also contributes to increased resistance to triazoles (108). A study showed that under in vitro conditions, A. fumigatus formed multicellular biofilms of polystyrene sheets that could resist the effects of antifungal drugs (109). Another study also showed an effect of itraconazole on hyphal germination and biofilm formation at an early stage, but no effect on mature biofilms, suggesting a predominance of resistant biofilms (110). Biofilms in the lungs are difficult to diagnose because they occur after mature biofilms form. In the adult stage, this tissue in the lungs develops into a more complex tissue with dense ECM and limited oxygen, which encourages further growth. This makes it increasingly difficult for immune cells to recognise and influence them. It also worsens when other microbial biofilms persist and are difficult to remove with antifungal drugs, particularly in cystic fibrosis (111). Therefore, a comprehensive analysis and understanding of Aspergillus biofilms is required to develop new and improved antifungal targets for the treatment of complex biofilm-related diseases (107).
Future directions
Azole resistance in environmental Aspergillus spp. is a matter of grave clinical concern as transfer of resistance from environment to clinical settings is inevitable. India needs to impose strict regulatory compliance to ensure regulated usage of pesticides in agricultural fields. Further studies are warranted to understand the level of transfer of resistance from the field to clinic. This can be undertaken in a state-wise study by mapping the mutations that are unique to the region. Additional studies focusing on the usage of azole fungicides and the presence of azole residues in developed environments are needed, because the amounts used or quantities present are not often measured or reported.
More surveillance, accurate data collection and comprehensive resistance surveillance programmes in agricultural ecosystems are needed to study the magnitude of the emerging problem of azole resistance. It will be very important to identify tipping points to ensure the agronomic use of fungicides without jeopardising their treatment goals. Research at the epidemiological level can uncover geographic differences in the emergence of resistance and help identify areas with high levels of resistance. Further, to overcome antifungal resistance in clinical settings, development of new antifungals with new drug target site is needed.
Acknowledgements
Authors would like to thank Amity University Uttar Pradesh for providing the infrastructure and facilities for research.
Disclosures
Conflict of interest: The authors declare no conflict of interest.
Financial support: Authors would like to thank CSIR for providing financial support in the form of Senior Research Fellowship (09/915(0013)/2018-EMR-I) to PS.
Authors’ contributions: PS performed literature search and drafted the manuscript; MV and SS performed literature search; SH critically analysed the manuscript; PV conceptualised the idea of review writing and critically analysed the manuscript.
References
- 1. Patterson TF, Kirkpatrick WR, White M, et al. Invasive aspergillosis. Disease spectrum, treatment practices, and outcomes. I3 Aspergillus Study Group Medicine (Baltimore). 2000;79(4):250-260. CrossRef PubMed
- 2. Lin SJ, Schranz J, Teutsch SM. Aspergillosis case-fatality rate: systematic review of the literature. Clin Infect Dis. 2001;32(3):358-366. CrossRef PubMed
- 3. Verweij PE, Chowdhary A, Melchers WJ, Meis JF. Azole resistance in A. fumigatus: can we retain the clinical use of mold-active antifungal azoles? Clin Infect Dis. 2016;62(3):362-368. CrossRef PubMed
- 4. Hagiwara D, Watanabe A, Kamei K, Goldman GH. Epidemiologi-cal and genomic landscape of azole resistance mechanisms in Aspergillus fungi. Front Microbiol. 2016;7:1382. CrossRef PubMed
- 5. Dabas Y, Xess I, Bakshi S, Mahapatra M, Seth R. Emergence of azole-resistant Aspergillus fumigatus from immunocompromised hosts in India. Antimicrob Agents Chemother. 2018;62(8):e02264-e17. CrossRef PubMed
- 6. Denning DW, Cadranel J, Beigelman-Aubry C, et al; European Society for Clinical Microbiology and Infectious Diseases and European Respiratory Society. Chronic pulmonary aspergillosis: rationale and clinical guidelines for diagnosis and management. Eur Respir J. 2016;47(1):45-68. CrossRef PubMed
- 7. Denning DW, Pleuvry A, Cole DC. Global burden of allergic bronchopulmonary aspergillosis with asthma and its complication chronic pulmonary aspergillosis in adults. Med Mycol. 2013;51(4):361-370. CrossRef PubMed
- 8. Armstead J, Morris J, Denning DW. Multi-country estimate of different manifestations of aspergillosis in cystic fibrosis. PLoS One. 2014;9(6):e98502. CrossRef PubMed
- 9. Denning DW. The ambitious ‘95-95 by 2025’ roadmap for the diagnosis and management of fungal diseases. Thorax. 2015;70(7):613-614. CrossRef PubMed
- 10. Kosmidis C, Denning DW. The clinical spectrum of pulmonary aspergillosis. Thorax. 2015;70(3):270-277. CrossRef PubMed
- 11. McCarthy MW, Walsh TJ. Special considerations for the diagnosis and treatment of invasive pulmonary aspergillosis. Expert Rev Respir Med. 2017;11(9):739-748. CrossRef PubMed
- 12. Komase Y, Kunishima H, Yamaguchi H, Ikehara M, Yamamoto T, Shinagawa T. Rapidly progressive invasive pulmonary aspergillosis in a diabetic man. J Infect Chemother. 2007;13(1):46-50. CrossRef PubMed
- 13. Yan X, Li M, Jiang M, Zou LQ, Luo F, Jiang Y. Clinical characteristics of 45 patients with invasive pulmonary aspergillosis: retrospective analysis of 1711 lung cancer cases. Cancer. 2009;115(21):5018-5025. CrossRef PubMed
- 14. Prattes J, Hoenigl M, Krause R, et al. Invasive aspergillosis in patients with underlying liver cirrhosis: a prospective cohort study. Med Mycol. 2017;55(8):803-812. CrossRef PubMed
- 15. Agarwal R, Denning DW, Chakrabarti A. Estimation of the burden of chronic and allergic pulmonary aspergillosis in India. PLoS One. 2014;9(12):e114745. CrossRef PubMed
- 16. Jabeen K, Farooqi J, Mirza S, Denning D, Zafar A. Serious fungal infections in Pakistan. Eur J Clin Microbiol Infect Dis. 2017;36(6):949-956. CrossRef PubMed
- 17. Gugnani HC, Denning DW, Rahim R, Sadat A, Belal M, Mahbub MS. Burden of serious fungal infections in Bangladesh. Eur J Clin Microbiol Infect Dis. 2017;36(6):993-997. CrossRef PubMed
- 18. Khwakhali US, Denning DW. Burden of serious fungal infections in Nepal. Mycoses. 2015;58(suppl 5):45-50. CrossRef PubMed
- 19. Jayasekera PI, Denning DW, Perera PD, Fernando A. The burden of serious fungal infections in Sri Lanka. Mycoses. 2013;56:103
- 20. Stevens DA, Moss RB, Kurup VP, et al; Participants in the Cystic Fibrosis Foundation Consensus Conference. Allergic bronchopulmonary aspergillosis in cystic fibrosis – state of the art: Cystic Fibrosis Foundation Consensus Conference. Clin Infect Dis. 2003;37(s3)(suppl 3):S225-S264. CrossRef PubMed
- 21. Al-Mobeireek AF, Al-Hedaithy SS, Alasali K, Al-Majed S, Joharjy I, Joharjy I; El-Rab MOGAD. Allergic bronchopulmonary mycosis in patients with asthma: period prevalence at a university hospital in Saudi Arabia. Respir Med. 2001;95(5):341-347. CrossRef PubMed
- 22. Lass-Flörl C. Triazole antifungal agents in invasive fungal infections: a comparative review. Drugs. 2011;71(18):2405-2419. CrossRef PubMed
- 23. Russell PE. A century of fungicide evolution. J Agric Sci. 2005;143(1):11-25. CrossRef
- 24. Gupta AK, Sauder DN, Shear NH. Antifungal agents: an overview. Part I. J Am Acad Dermatol. 1994;30(5 Pt 1):677-698. CrossRef PubMed
- 25. Heeres J, Backx LJ, Mostmans JH, Van Cutsem J. Antimycotic imidazoles. Part 4. Synthesis and antifungal activity of ketoconazole, a new potent orally active broad-spectrum antifungal agent. J Med Chem. 1979;22(8):1003-1005. CrossRef PubMed
- 26. Johnson LB, Kauffman CA. Voriconazole: a new triazole antifungal agent. Clin Infect Dis. 2003;36(5):630-637. CrossRef PubMed
- 27. Maertens JA. History of the development of azole derivatives. Clin Microbiol Infect. 2004;10(s1)(suppl 1):1-10. CrossRef PubMed
- 28. Scott LJ, Simpson D. Voriconazole: a review of its use in the management of invasive fungal infections. Drugs. 2007;67(2):269-298. CrossRef PubMed
- 29. Gothard P, Rogers TR. Voriconazole for serious fungal infections. Int J Clin Pract. 2004;58(1):74-80. CrossRef PubMed
- 30. Pasqualotto AC, Thiele KO, Goldani LZ. Novel triazole antifungal drugs: focus on isavuconazole, ravuconazole and albaconazole. Curr Opin Investig Drugs. 2010;11(2):165-174. PubMed
- 31. Allen D, Wilson D, Drew R, Perfect J. Azole antifungals: 35 years of invasive fungal infection management. Expert Rev Anti Infect Ther. 2015;13(6):787-798. CrossRef PubMed
- 32. Snelders E, Karawajczyk A, Schaftenaar G, Verweij PE, Melchers WJ. Azole resistance profile of amino acid changes in Aspergillus fumigatus CYP51A based on protein homology modeling. Antimicrob Agents Chemother. 2010;54(6):2425-2430. CrossRef PubMed
- 33. Georgopapadakou NH, Walsh TJ. Antifungal agents: chemotherapeutic targets and immunologic strategies. Antimicrob Agents Chemother. 1996;40(2):279-291. CrossRef PubMed
- 34. White TC, Marr KA, Bowden RA. Clinical, cellular, and molecular factors that contribute to antifungal drug resistance. Clin Microbiol Rev. 1998;11(2):382-402. CrossRef PubMed
- 35. Koltin Y, Hitchcock CA. The search for new triazole antifungal agents. Curr Opin Chem Biol. 1997;1(2):176-182. CrossRef PubMed
- 36. Maschmeyer G, Haas A, Cornely OA. Invasive aspergillosis: epidemiology, diagnosis and management in immunocompromised patients. Drugs. 2007;67(11):1567-1601. CrossRef PubMed
- 37. Walsh TJ, Anaissie EJ, Denning DW, et al; Infectious Diseases Society of America. Treatment of aspergillosis: clinical practice guidelines of the Infectious Diseases Society of America. Clin Infect Dis. 2008;46(3):327-360. CrossRef PubMed
- 38. Howard SJ, Pasqualotto AC, Denning DW. Azole resistance in allergic bronchopulmonary aspergillosis and Aspergillus bronchitis. Clin Microbiol Infect. 2010;16(6):683-688. CrossRef PubMed
- 39. Mukherjee PK, Sheehan D, Puzniak L, Schlamm H, Ghannoum MA. Echinocandins: are they all the same? J Chemother. 2011;23(6):319-325. CrossRef PubMed
- 40. Azevedo MM, Faria-Ramos I, Cruz LC, Pina-Vaz C, Rodrigues AG. Genesis of azole antifungal resistance from agriculture to clinical settings. J Agric Food Chem. 2015;63(34):7463-7468. CrossRef PubMed
- 41. Morton V, Staub T. A Short History of Fungicides. APSnet Features. doi: 10.1094/APSnet Feature-2008-0308. Online
- 42. Price CL, Parker JE, Warrilow AG, Kelly DE, Kelly SL. Azole fungicides – understanding resistance mechanisms in agricultural fungal pathogens. Pest Manag Sci. 2015;71(8):1054-1058. CrossRef PubMed
- 43. Singh N, Dureja P. Persistence of hexaconazole, a triazole fungicide in soils. J Environ Sci Health B. 2000;35(5):549-558. CrossRef PubMed
- 44. FRAC Code List. Fungal control agents sorted by cross resistance pattern and mode of action. Fungicide Resistance Action Committee; 2019.
- 45. Messer SA, Jones RN, Fritsche TR. International surveillance of Candida spp. and Aspergillus spp.: report from the SENTRY Antimicrobial Surveillance Program (2003). J Clin Microbiol. 2006;44(5):1782-1787. CrossRef PubMed
- 46. Gomez-Lopez A, Garcia-Effron G, Mellado E, Monzon A, Rodriguez-Tudela JL, Cuenca-Estrella M. In vitro activities of three licensed antifungal agents against Spanish clinical isolates of Aspergillus spp. Antimicrob Agents Chemother. 2003;47(10):3085-3088. CrossRef PubMed
- 47. Lelièvre L, Groh M, Angebault C, Maherault AC, Didier E, Bougnoux ME. Azole resistant Aspergillus fumigatus: an emerging problem. Med Mal Infect. 2013;43(4):139-145. CrossRef PubMed
- 48. Mellado E, Garcia-Effron G, Alcázar-Fuoli L, Cuenca-Estrella M, Rodriguez-Tudela JL. Substitutions at methionine 220 in the 14α-sterol demethylase (Cyp51A) of Aspergillus fumigatus are responsible for resistance in vitro to azole antifungal drugs. Antimicrob Agents Chemother. 2004;48(7):2747-2750. CrossRef PubMed
- 49. Mellado E, Garcia-Effron G, Alcázar-Fuoli L, et al. A new Aspergillus fumigatus resistance mechanism conferring in vitro cross-resistance to azole antifungals involves a combination of cyp51A alterations. Antimicrob Agents Chemother. 2007;51(6):1897-1904. CrossRef PubMed
- 50. Alanio A, Sitterlé E, Liance M, et al. Low prevalence of resistance to azoles in Aspergillus fumigatus in a French cohort of patients treated for haematological malignancies. J Antimicrob Chemother. 2011;66(2):371-374. CrossRef PubMed
- 51. Mortensen KL, Jensen RH, Johansen HK, et al. Aspergillus species and other molds in respiratory samples from patients with cystic fibrosis: a laboratory-based study with focus on Aspergillus fumigatus azole resistance. J Clin Microbiol. 2011;49(6):2243-2251. CrossRef PubMed
- 52. Escribano P, Recio S, Peláez T, Bouza E, Guinea J. Aspergillus fumigatus strains with mutations in the cyp51A gene do not always show phenotypic resistance to itraconazole, voriconazole, or posaconazole. Antimicrob Agents Chemother. 2011;55(5):2460-2462. CrossRef PubMed
- 53. Jeurissen A, Cooreman S, Van Kerckhoven W, et al. Invasive pulmonary aspergillosis due to a multi-azole resistant Aspergillus fumigatus. Acta Clin Belg. 2012;67(1):46-48. PubMed
- 54. Alastruey-Izquierdo A, Mellado E, Peláez T, et al; FILPOP Study Group. Population-based survey of filamentous fungi and antifungal resistance in Spain (FILPOP Study). Antimicrob Agents Chemother. 2013;57(7):3380-3387. CrossRef PubMed
- 55. Steinmann J, Hamprecht A, Vehreschild MJ, et al. Emergence of azole-resistant invasive aspergillosis in HSCT recipients in Germany. J Antimicrob Chemother. 2015;70(5):1522-1526. CrossRef PubMed
- 56. van der Linden JW, Arendrup MC, Warris A, et al. Prospective multicenter international surveillance of azole resistance in Aspergillus fumigatus. Emerg Infect Dis. 2015;21(6):1041-1044. CrossRef PubMed
- 57. Lavergne RA, Morio F, Favennec L, et al. First description of azole-resistant Aspergillus fumigatus due to TR46/Y121F/T289A mutation in France. Antimicrob Agents Chemother. 2015;59(7):4331-4335. CrossRef PubMed
- 58. Brillowska-Dąbrowska A, Mroczyńska M, Nawrot U, Włodarczyk K, Kurzyk E. Examination of cyp51A and cyp51B expression level of the first Polish azole resistant clinical Aspergillus fumigatus isolate. Acta Biochim Pol. 2015;62(4):837-839. CrossRef PubMed
- 59. Lazzarini C, Esposto MC, Prigitano A, Cogliati M, De Lorenzis G, Tortorano AM. Azole resistance in A. fumigatus clinical isolates from an Italian culture collection. Antimicrob Agents Chemother. 2015;60(1):682-685. CrossRef PubMed
- 60. Pelaez T, Monteiro MC, Garcia-Rubio R, Bouza E, Gomez-Lopez A, Mellado E. First detection of Aspergillus fumigatus azole-resistant strain due to Cyp51A TR46/Y121F/T289A in an azole-naive patient in Spain. New Microbes New Infect. 2015;6:33-34. CrossRef PubMed
- 61. Özmerdiven GE, Ak S, Ener B, et al. First determination of azole resistance in Aspergillus fumigatus strains carrying the TR34/L98H mutations in Turkey. J Infect Chemother. 2015;21(8):581-586. CrossRef PubMed
- 62. Jensen RH, Hagen F, Astvad KM, Tyron A, Meis JF, Arendrup MC. Azole-resistant Aspergillus fumigatus in Denmark: a laboratory-based study on resistance mechanisms and genotypes. Clin Microbiol Infect. 2016 Jun;22(6):570.e1-e9. CrossRef PubMed
- 63. Lavergne RA, Chouaki T, Hagen F, et al. Home environment as a source of life-threatening azole-resistant A. fumigatus in immunocompromised patients. Clin Infect Dis. 2017;64(1):76-78. CrossRef PubMed
- 64. Prigitano A, Esposto MC, Biffi A, et al. Triazole resistance in Aspergillus fumigatus isolates from patients with cystic fibrosis in Italy. J Cyst Fibros. 2017;16(1):64-69. CrossRef PubMed
- 65. Abdolrasouli A, Petrou MA, Park H, et al. Surveillance for azole-resistant Aspergillus fumigatus in a centralized diagnostic mycology service, London, United Kingdom, 1998-2017. Front Microbiol. 2018;9:2234. CrossRef PubMed
- 66. Wu CJ, Liu WL, Lai CC, et al. Multicenter study of azole-resistant Aspergillus fumigatus clinical isolates, Taiwan. Emerg Infect Dis. 2020;26(4):804-806. CrossRef PubMed
- 67. Chen J, Li H, Li R, Bu D, Wan Z. Mutations in the cyp51A gene and susceptibility to itraconazole in Aspergillus fumigatus serially isolated from a patient with lung aspergilloma. J Antimicrob Chemother. 2005;55(1):31-37. CrossRef PubMed
- 68. Hsueh PR, Lau YJ, Chuang YC, et al. Antifungal susceptibilities of clinical isolates of Candida species, Cryptococcus neoformans, and Aspergillus species from Taiwan: surveillance of multicenter antimicrobial resistance in Taiwan program data from 2003. Antimicrob Agents Chemother. 2005;49(2):512-517. CrossRef PubMed
- 69. Bueid A, Howard SJ, Moore CB, et al. Azole antifungal resistance in Aspergillus fumigatus: 2008 and 2009. J Antimicrob Chemother. 2010;65(10):2116-2118. CrossRef PubMed
- 70. Howard SJ, Cerar D, Anderson MJ, et al. Frequency and evolution of azole resistance in Aspergillus fumigatus associated with treatment failure. Emerg Infect Dis. 2009;15(7):1068-1076. CrossRef PubMed
- 71. Meis JF, Chowdhary A, Rhodes JL, Fisher MC, Verweij PE. Clinical implications of globally emerging azole resistance in Aspergillus fumigatus. Philos Trans R Soc Lond B Biol Sci. 2016;371(1709):20150460. CrossRef PubMed
- 72. Bunskoek PE, Seyedmousavi S, Gans SJ, et al. Successful treatment of azole-resistant invasive aspergillosis in a bottlenose dolphin with high-dose posaconazole. Med Mycol Case Rep. 2017;16:16-19. CrossRef PubMed
- 73. Hof H. Critical annotations to the use of azole antifungals for plant protection. Antimicrob Agents Chemother. 2001;45(11):2987-2990. CrossRef PubMed
- 74. Snelders E, Camps SM, Karawajczyk A, et al. Triazole fungicides can induce cross-resistance to medical triazoles in Aspergillus fumigatus. PLoS One. 2012;7(3):e31801. CrossRef PubMed
- 75. Chowdhary A, Kathuria S, Xu J, et al. Clonal expansion and emergence of environmental multiple-triazole-resistant Aspergillus fumigatus strains carrying the TR₃₄/L98H mutations in the cyp51A gene in India. PLoS One. 2012;7(12):e52871. CrossRef PubMed
- 76. Mortensen KL, Mellado E, Lass-Flörl C, Rodriguez-Tudela JL, Johansen HK, Arendrup MC. Environmental study of azole-resistant Aspergillus fumigatus and other aspergilli in Austria, Denmark, and Spain. Antimicrob Agents Chemother. 2010;54(11):4545-4549. CrossRef PubMed
- 77. Chowdhary A, Kathuria S, Xu J, Meis JF. Emergence of azole-resistant Aspergillus fumigatus strains due to agricultural azole use creates an increasing threat to human health. PLoS Pathog. 2013;9(10):e1003633. CrossRef PubMed
- 78. Verweij PE, Mellado E, Melchers WJ. Multiple-triazole-resistant aspergillosis. N Engl J Med. 2007;356(14):1481-1483. CrossRef PubMed
- 79. Van der Linden JW, Camps SM, Kampinga GA, et al. Aspergillosis due to voriconazole highly resistant A. fumigatus and recovery of genetically related resistant isolates from domestic homes. Clin Infect Dis. 2013;57(4):513-520. CrossRef PubMed
- 80. Thind TS. Changing cover of fungicide umbrella in crop protection. Indian Phytopathol. 2007;60:421-433.
- 81. Snelders E, Huis in t Veld RA, Rijs AJ, Kema GH, Melchers WJ, Verweij PE. Possible environmental origin of resistance of A. fumigatus to medical triazoles. Appl Environ Microbiol. 2009;75(12):4053-4057. CrossRef PubMed
- 82. Zhang J, Snelders E, Zwaan BJ, et al. A novel environmental azole resistance mutation in A. fumigatus and a possible role of sexual reproduction in its emergence. MBio. 2017;8(3):e00791-e17. CrossRef PubMed
- 83. Badali H, Vaezi A, Haghani I, et al. Environmental study of azole-resistant Aspergillus fumigatus with TR34/L98H mutations in the cyp51A gene in Iran. Mycoses. 2013;56(6):659-663. CrossRef PubMed
- 84. Ahmad S, Khan Z, Hagen F, Meis JF. Occurrence of triazole-resistant Aspergillus fumigatus with TR34/L98H mutations in outdoor and hospital environment in Kuwait. Environ Res. 2014;133:20-26. CrossRef PubMed
- 85. Chowdhary A, Sharma C, Hagen F, Meis JF. Exploring azole antifungal drug resistance in Aspergillus fumigatus with special reference to resistance mechanisms. Future Microbiol. 2014;9(5):697-711. CrossRef PubMed
- 86. van der Linden JW, Snelders E, Kampinga GA, et al. Clinical implications of azole resistance in Aspergillus fumigatus, The Netherlands, 2007-2009. Emerg Infect Dis. 2011;17(10):1846-1854. CrossRef PubMed
- 87. Dauchy C, Bautin N, Nseir S, et al. Emergence of Aspergillus fumigatus azole resistance in azole-naïve patients with chronic obstructive pulmonary disease and their homes. Indoor Air. 2018;28(2):298-306. CrossRef PubMed
- 88. Lescar J, Meyer I, Akshita K, et al. Aspergillus fumigatus harbouring the sole Y121F mutation shows decreased susceptibility to voriconazole but maintained susceptibility to itraconazole and posaconazole. J Antimicrob Chemother. 2014;69(12):3244-3247. CrossRef PubMed
- 89. Sharma C, Hagen F, Moroti R, Meis JF, Chowdhary A. Triazole-resistant Aspergillus fumigatus harbouring G54 mutation: is it de novo or environmentally acquired? J Glob Antimicrob Resist. 2015;3(2):69-74. CrossRef PubMed
- 90. Wang HC, Huang JC, Lin YH, Chen YH. Prevalence, mechanisms, and genetic relatedness of the human pathogenic fungus A. fumigatus sex inhibiting resistance to medical azoles in the environment of Taiwan. Appl Environ Microbiol. 2008;20:270-280.
- 91. Jeanvoine A, Rocchi S, Reboux G, Crini N, Crini G, Millon L. Azole-resistant Aspergillus fumigatus in sawmills of Eastern France. J Appl Microbiol. 2017;123(1):172-184. CrossRef PubMed
- 92. Alvarez-Moreno C, Lavergne RA, Hagen F, Morio F, Meis JF, Le Pape P. Azole-resistant Aspergillus fumigatus harboring TR34/L98H, TR46/Y121F/T289A and TR53 mutations related to flower fields in Colombia. Sci Rep. 2017;7(1):45631. CrossRef PubMed
- 93. Chowdhary A, Sharma C, Kathuria S, Hagen F, Meis JF. Prevalence and mechanism of triazole resistance in Aspergillus fumigatus in a referral chest hospital in Delhi, India and an update of the situation in Asia. Front Microbiol. 2015;6:428. CrossRef PubMed
- 94. Hoda S, Agarwal H, Ahluwalia SK, Vermani M, Vijayaraghavan P. Antifungal resistance analysis of environmental isolates of Aspergillus in North India. J Pure Appl Microbiol. 2019;13(1):385-392. CrossRef
- 95. Mann PA, Parmegiani RM, Wei SQ, et al. Mutations in Aspergillus fumigatus resulting in reduced susceptibility to posaconazole appear to be restricted to a single amino acid in the cytochrome P450 14α-demethylase. Antimicrob Agents Chemother. 2003;47(2):577-581. CrossRef PubMed
- 96. Tashiro M, Izumikawa K, Hirano K, et al. Correlation between triazole treatment history and susceptibility in clinically isolated Aspergillus fumigatus. Antimicrob Agents Chemother. 2012;56(9):4870-4875. CrossRef PubMed
- 97. Chowdhary A, Sharma C, van den Boom M, et al. Multi-azole-resistant Aspergillus fumigatus in the environment in Tanzania. J Antimicrob Chemother. 2014;69(11):2979-2983. CrossRef PubMed
- 98. Rajendran R, Mowat E, McCulloch E, et al. Azole resistance of Aspergillus fumigatus biofilms is partly associated with efflux pump activity. Antimicrob Agents Chemother. 2011;55(5):2092-2097. CrossRef PubMed
- 99. Tekaia F, Latgé JP. Aspergillus fumigatus: saprophyte or pathogen? Curr Opin Microbiol. 2005;8(4):385-392. CrossRef PubMed
- 100. Wesenberg D, Kyriakides I, Agathos SN. White-rot fungi and their enzymes for the treatment of industrial dye effluents. Biotechnol Adv. 2003;22(1-2):161-187. CrossRef PubMed
- 101. Dagenais TR, Keller NP. Pathogenesis of Aspergillus fumigatus in invasive aspergillosis. Clin Microbiol Rev. 2009;22(3):447-465. CrossRef PubMed
- 102. Godeau C, Reboux G, Scherer E, et al. Azole-resistant Aspergillus fumigatus in the hospital: surveillance from flower beds to corridors. Am J Infect Control. 2020;48(6):702-704. CrossRef PubMed
- 103. Gonzalez-Jimenez I, Lucio J, Menéndez-Fraga MD, Mellado E, Peláez T. Hospital environment as a source of azole-resistant Aspergillus fumigatus strains with TR34/L98H and G448S Cyp51A mutations. J Fungi (Basel). 2021;7(1):22. CrossRef PubMed
- 104. Bowyer P, Moore CB, Rautemaa R, Denning DW, Richardson MD. Azole antifungal resistance today: focus on Aspergillus. Curr Infect Dis Rep. 2011;13(6):485-491. CrossRef PubMed
- 105. Mitchell KF, Zarnowski R, Andes DR. The extracellular matrix of fungal biofilms. Adv Exp Med Biol. 2016;931:21-35. CrossRef PubMed
- 106. Subroto E, van Neer J, Valdes I, de Cock H. Growth of Aspergillus fumigatus in biofilms in comparison to Candida albicans. J Fungi (Basel). 2022;8(1):48. CrossRef PubMed
- 107. Kaur S, Singh S. Biofilm formation by Aspergillus fumigatus. Med Mycol. 2014;52(1):2-9. PubMed
- 108. Blankenship JR, Mitchell AP. How to build a biofilm: a fungal perspective. Curr Opin Microbiol. 2006;9(6):588-594. CrossRef PubMed
- 109. Mowat E, Butcher J, Lang S, Williams C, Ramage G. Development of a simple model for studying the effects of antifungal agents on multicellular communities of Aspergillus fumigatus. J Med Microbiol. 2007;56(Pt 9):1205-1212. CrossRef PubMed
- 110. Liu M, Zheng H, Zeng R, Liang G, Zheng N, Liu W. Effects of itraconazole and micafungin on Aspergillus fumigatus biofilms. Mycopathologia. 2021;186(3):387-397. CrossRef PubMed
- 111. Morelli KA, Kerkaert JD, Cramer RA. Aspergillus fumigatus biofilms: toward understanding how growth as a multicellular network increases antifungal resistance and disease progression. PLoS Pathog. 2021;17(8):e1009794. CrossRef PubMed